Breast Cancer Research

Breast Cancer Risk Factors
Breast Cancer Research is presenting our Retrospective Collection on "Breast Cancer Risk Factors." Celebrating 'Breast Cancer Awareness Month (1 October- 31 October)', with this Collection, we aim to gain valuable insights into the multifaceted aspects of breast cancer risk to promote awareness, prevention, and early detection.
NEW CROSS-JOURNAL COLLECTIONS Find out more by clicking the links below:
Artif icial Intelligence in Breast Imaging PDGFB in Br east Cancer Initiation,Progression, and Metastasis
Aims and scope
- Most accessed
Characterization of tumor-infiltrating lymphocytes and their spatial distribution in triple-negative breast cancer
Authors: Eunkyung Han, Hye Yeon Choi, Hyun Jung Kwon, Yul Ri Chung, Hee-Chul Shin, Eun-Kyu Kim, Koung Jin Suh, Se Hyun Kim, Jee Hyun Kim and So Yeon Park
Alcohol cessation and breast cancer risk stratified by hormone receptor status
Authors: Mary Beth Terry, Dallas R. English, Jo L. Freudenheim, Béatrice Lauby-Secretan and Susan M. Gapstur
Multimodal genome-wide survey of progressing and non-progressing breast ductal carcinoma in-situ
Authors: Marija Debeljak, Soonweng Cho, Bradley M. Downs, Michael Considine, Brittany Avin-McKelvey, Yongchun Wang, Phillip N. Perez, William E. Grizzle, Katherine A. Hoadley, Charles F. Lynch, Brenda Y. Hernandez, Paul J. van Diest, Wendy Cozen, Ann S. Hamilton, Debra Hawes, Edward Gabrielson…
Image analysis-based identification of high risk ER-positive, HER2-negative breast cancers
Authors: Dong Neuck Lee, Yao Li, Linnea T. Olsson, Alina M. Hamilton, Benjamin C. Calhoun, Katherine A. Hoadley, J. S. Marron and Melissa A. Troester
Social vulnerability is associated with advanced breast cancer presentation and all-cause mortality: a retrospective cohort study
Authors: Kayla A. Councell, Ann M. Polcari, Rachel Nordgren, Ted A. Skolarus, Andrew J. Benjamin and Sarah P. Shubeck
Most recent articles RSS
View all articles
Serum thymidine kinase 1 activity as a pharmacodynamic marker of cyclin-dependent kinase 4/6 inhibition in patients with early-stage breast cancer receiving neoadjuvant palbociclib
Authors: Nusayba Bagegni, Shana Thomas, Ning Liu, Jingqin Luo, Jeremy Hoog, Donald W. Northfelt, Matthew P. Goetz, Andres Forero, Mattias Bergqvist, Jakob Karen, Magnus Neumüller, Edward M. Suh, Zhanfang Guo, Kiran Vij, Souzan Sanati, Matthew Ellis…
Choosing the right cell line for breast cancer research
Authors: Deborah L Holliday and Valerie Speirs
Triple-negative breast cancer molecular subtyping and treatment progress
Authors: Li Yin, Jiang-Jie Duan, Xiu-Wu Bian and Shi-cang Yu
Breast asymmetry and predisposition to breast cancer
Authors: Diane Scutt, Gillian A Lancaster and John T Manning
Critical research gaps and translational priorities for the successful prevention and treatment of breast cancer
Authors: Suzanne A Eccles, Eric O Aboagye, Simak Ali, Annie S Anderson, Jo Armes, Fedor Berditchevski, Jeremy P Blaydes, Keith Brennan, Nicola J Brown, Helen E Bryant, Nigel J Bundred, Joy M Burchell, Anna M Campbell, Jason S Carroll, Robert B Clarke, Charlotte E Coles…
Most accessed articles RSS
Editor-in-Chief
Lewis Chodosh , University of Pennsylvania, USA
Trending in the Media
Click here to see the most popular articles published in Breast Cancer Research in the past three months.
BCR's 20th Anniversary
20 years ago Breast Cancer Research published its first articles with BMC. Well-respected in the field, the journal has continually placed in the first quartile of the ‘Oncology’ category of Journal Citation Reports. Over the past decade, Breast Cancer Research (BCR) has also become the highest ranked breast cancer focused title in the field.
Look back at the journal’s milestone achievements and article highlights .
Featured Review - Artificial intelligence in mammographic phenotyping of breast cancer risk: a narrative review
In this review, we provide a useful reference for AI researchers investigating image-based breast cancer risk assessment while indicating key priorities and challenges that, if properly addressed, could accelerate the implementation of AI-assisted risk stratification to future refine and individualize breast cancer screening strategies.
Springer Nature Oncology Portfolio
Discover the range of academic oncology titles at Springer Nature here .
- Adolescent and Young Adult Cancer
- Bile Duct Cancer
- Bladder Cancer
- Brain Cancer
- Breast Cancer
- Cervical Cancer
- Childhood Cancer
- Colorectal Cancer
- Endometrial Cancer
- Esophageal Cancer
- Head and Neck Cancer
- Kidney Cancer
- Liver Cancer
- Lung Cancer
- Mouth Cancer
- Mesothelioma
- Multiple Myeloma
- Neuroendocrine Tumors
- Ovarian Cancer
- Pancreatic Cancer
- Prostate Cancer
- Skin Cancer/Melanoma
- Stomach Cancer
- Testicular Cancer
- Throat Cancer
- Thyroid Cancer
- Prevention and Screening
- Diagnosis and Treatment
- Research and Clinical Trials
- Survivorship

Request an appointment at Mayo Clinic

Advances in breast cancer research: Decreasing the need for chemotherapy and reducing the intensity of radiation therapy
Share this:.

By Kelley Luckstein
Lifesaving cancer therapies can cause serious side effects, both immediately and later in life. "It is essential to continue to study innovative approaches that will eradicate the disease but won't diminish the quality of life for patients diagnosed with cancer," says Roberto Leon-Ferre, M.D. , a breast medical oncologist at Mayo Clinic. With breast cancer rates rising among younger people, the need for treatments that provide excellent outcomes with fewer side effects is only increasing.
Mayo Clinic Comprehensive Cancer Center experts highlight breast cancer research that may reduce or eliminate the need for chemotherapy and endocrine treatments that may allow for shorter and less intense radiation therapy courses.
Prognostic Biomarker Identified in Triple-Negative Breast Cancer: Potential to Reduce Chemotherapy Use
Dr. Leon-Ferre's research team recently identified a prognostic biomarker in patients diagnosed with triple-negative breast cancer (TNBC) , a type of breast cancer that does not have estrogen or progesterone receptors and that does not have excessive expression of HER2. The researchers found that patients with high levels of naturally existing immune cells called tumor-infiltrating lymphocytes (TILs) within their tumors may have a lower risk of cancer recurrence and better survival rates, even when chemotherapy is not administered.
"This is an important finding because triple-negative breast cancer is a particularly aggressive form of breast cancer that does not respond to hormonal therapies or drugs targeting the HER2 protein," says Dr. Leon-Ferre. "This means treatment options are more limited, often more intense and harsher for patients. However, some patients with TNBC can be cured without chemotherapy, and the key is identifying who those patients are ahead of time." He adds that future research will explore whether TILs can be used to decide who may need and may not need chemotherapy after a TNBC diagnosis.
Study Explores Necessity of Chemotherapy in Premenopausal Women with Intermediate-Risk Breast Cancer
In a separate ongoing trial, Tufia Haddad, M.D. , breast medical oncologist at Mayo Clinic, is co-chair of a study comparing two treatment regimens in premenopausal women with intermediate-risk breast cancer: one group with chemotherapy plus ovarian suppression and hormone therapy, and another group without chemotherapy but with ovarian suppression and hormone therapy. The study aims to determine whether chemotherapy's benefits come from its ability to suppress ovarian function (thereby reducing estrogen production) or from its direct cancer-fighting effects. "The results could determine if chemotherapy is necessary or if ovarian suppression and hormone therapy alone are enough in this group of patients, sparing them from the side effects of chemotherapy," says Dr. Haddad.
Endoxifen: A Potent Therapy for Estrogen-Receptor-Positive Breast Cancer Explored by Mayo Clinic
Matthew Goetz, M.D. , a breast medical oncologist and director of the Mayo Clinic Breast Cancer Specialized Programs of Research Excellence (SPORE) , and the Erivan K. Haub Family Professor of Cancer Research Honoring Richard F. Emslander, M.D., has spent more than a decade studying the use of endoxifen for the treatment of patients with estrogen-receptor-positive breast cancer (ER-positive breast cancer or ER+), the most common subtype of breast cancer.
Endoxifen, the most potent byproduct of tamoxifen, stops the growth of estrogen-dependent cancer cells and blocks protein kinase C, which may contribute to the aggressiveness of some cancers. Premenopausal patients with ER+ breast cancer are treated with ovarian function suppression and aromatase inhibitors to lower estrogen levels and slow cancer growth. While effective, this regimen causes significant side effects from deep estrogen suppression. Dr. Goetz's SPORE-funded study explores whether endoxifen alone can eradicate cancer cells, potentially eliminating the need for more toxic treatments like chemotherapy. He is also studying the role of protein kinase C in ER+ breast cancer.
Mayo Clinic Study Finds Shortened and Less Intense Radiation Therapy Effective for Breast Cancer Patients
Radiation therapy can be an essential part of a person's breast cancer treatment plan; however, it can lead to changes in the appearance of the skin, swelling, pain and other complications. These side effects can be detrimental to a patient's quality of life. In a recent study , Mayo Clinic breast cancer researchers found they can safely reduce the intensity and shorten the course of radiation treatment to three days for select patients with breast cancer following their lumpectomy.
"Normally, this treatment takes five to 15 days and can sometimes cause unwanted side effects, including changes in how the breast looks. However, this new method administers a lower dose of radiation over just three sessions using either photons, protons or brachytherapy," says Robert Mutter, M.D. , a Mayo Clinic radiation oncologist and lead author of the study. "The new personalized approach was well-tolerated by the patients and resulted in excellent cancer control with better long-term aesthetic results compared to what has previously been reported with longer treatments."
Collectively, this research is leading to innovative approaches and helping clinicians and patients make informed treatment decisions, potentially sparing patients from some of the more severe side effects of chemotherapy, endocrine therapy and radiation.
Learn more about breast cancer and find a clinical trial at Mayo Clinic.
Join the Breast Cancer Support Group on Mayo Clinic Connect , an online community moderated by Mayo Clinic for patients and caregivers.
Also, read these articles:
- Breast cancer types: What your type means
- New study finds triple-negative breast cancer tumors with an increase in immune cells have lower risk of recurrence after surgery
- Understanding triple-negative breast cancer and its treatment
- The 4 types of systemic therapy for breast cancer
- Mayo Clinic study reveals proton beam therapy may shorten breast cancer treatment
A version of this article was originally published on the Mayo Clinic News Network .
Online Help
Our 24/7 cancer helpline provides information and answers for people dealing with cancer. We can connect you with trained cancer information specialists who will answer questions about a cancer diagnosis and provide guidance and a compassionate ear.

Chat live online
Select the Live Chat button at the bottom of the page

Call us at 1-800-227-2345
Available any time of day or night
Our highly trained specialists are available 24/7 via phone and on weekdays can assist through online chat. We connect patients, caregivers, and family members with essential services and resources at every step of their cancer journey. Ask us how you can get involved and support the fight against cancer. Some of the topics we can assist with include:
- Referrals to patient-related programs or resources
- Donations, website, or event-related assistance
- Tobacco-related topics
- Volunteer opportunities
- Cancer Information
For medical questions, we encourage you to review our information with your doctor.
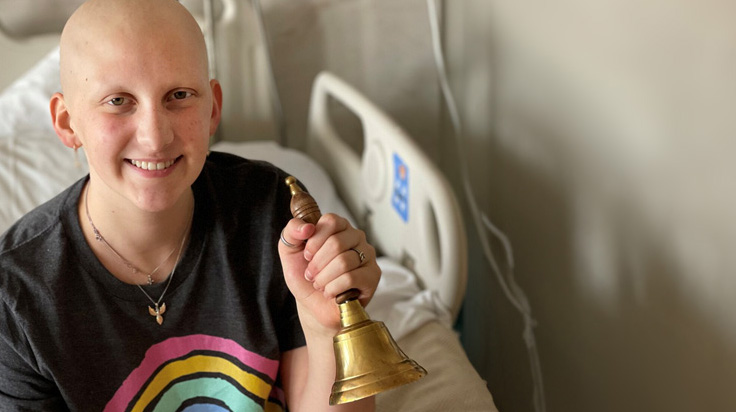
Donate today to support our lifesaving efforts.
Your gift enables us to support people with cancer and fund research breakthroughs.
The amount must be greater than or equal to $5
Your gift is 100% tax deductible
Breast Cancer
- What Is Breast Cancer?
- What Causes Breast Cancer?
- Ductal Carcinoma in Situ (DCIS)
- Invasive Breast Cancer (IDC/ILC)
- Triple-negative Breast Cancer
- Inflammatory Breast Cancer
- Angiosarcoma of the Breast
- Paget Disease of the Breast
- Phyllodes Tumors
- Key Statistics for Breast Cancer
What’s New in Breast Cancer Research?
- Breast Cancer Risk Factors You Cannot Change
- Lifestyle-related Breast Cancer Risk Factors
- Factors with Unclear Effects on Breast Cancer Risk
- Disproven or Controversial Breast Cancer Risk Factors
- Can I Lower My Risk of Breast Cancer?
- Genetic Counseling and Testing for Breast Cancer Risk
- Deciding Whether to Use Medicine to Reduce Breast Cancer Risk
- Tamoxifen and Raloxifene for Lowering Breast Cancer Risk
- Aromatase Inhibitors for Lowering Breast Cancer Risk
- Preventive Surgery to Reduce Breast Cancer Risk
- American Cancer Society Recommendations for the Early Detection of Breast Cancer
- Mammogram Basics
- Tips for Getting a Mammogram
- What Does the Doctor Look for on a Mammogram?
- Getting Called Back After a Mammogram
- Understanding Your Mammogram Report
- Breast Density and Your Mammogram Report
- Limitations of Mammograms
- Mammograms After Breast Cancer Surgery
- Mammograms for Women with Breast Implants
- Breast Ultrasound
- Newer and Experimental Breast Imaging Tests
- Breast Cancer Signs and Symptoms
- Fine Needle Aspiration (FNA) of the Breast
- Core Needle Biopsy of the Breast
- Surgical Breast Biopsy
- Questions to Ask Before a Breast Biopsy
- Finding Breast Cancer During Pregnancy
- Breast Cancer Grades
- Breast Cancer Ploidy and Cell Proliferation
- Breast Cancer Hormone Receptor Status
- Breast Cancer HER2 Status
- Breast Cancer Gene Expression Tests
- Other Breast Cancer Gene, Protein, and Blood Tests
- Imaging Tests to Find Out if Breast Cancer Has Spread
- Breast Cancer Stages
- Breast Cancer Survival Rates
- Questions to Ask Your Doctor About Breast Cancer
- Breast-conserving Surgery (Lumpectomy)
- Lymph Node Surgery for Breast Cancer
- Exercises After Breast Cancer Surgery
- Radiation for Breast Cancer
- Chemotherapy for Breast Cancer
- Hormone Therapy for Breast Cancer
- Targeted Drug Therapy for Breast Cancer
- Immunotherapy for Breast Cancer
- Treatment of Ductal Carcinoma in Situ (DCIS)
- Treatment of Breast Cancer Stages I-III
- Treatment of Stage IV (Metastatic) Breast Cancer
- Treatment of Recurrent Breast Cancer
- Treatment of Triple-negative Breast Cancer
- Treatment of Inflammatory Breast Cancer
- Treating Breast Cancer During Pregnancy
- Should I Get Breast Reconstruction Surgery?
- Breast Reconstruction Alternatives
- Breast Reconstruction After Breast-conserving Surgery
- Breast Reconstruction Using Implants
- Breast Reconstruction Using Your Own Tissues (Flap Procedures)
- Reconstructing the Nipple and Areola After Breast Surgery
- Questions to Ask Your Surgeon About Breast Reconstruction
- Preparing for Breast Reconstruction Surgery
- What to Expect After Breast Reconstruction Surgery
- Follow-up Care After Breast Cancer Treatment
- Can I Lower My Risk of Breast Cancer Progressing or Coming Back?
- Body Image and Sexuality After Breast Cancer
- Pregnancy After Breast Cancer
- Menopausal Hormone Therapy After Breast Cancer
- Second Cancers After Breast Cancer
- If You Have Breast Cancer
- Fibrosis and Simple Cysts
- Hyperplasia (Ductal or Lobular)
- Lobular Carcinoma in Situ (LCIS)
- Fibroadenomas
- Intraductal Papillomas
- Fat Necrosis and Oil Cysts
- Duct Ectasia
- Radial Scars and Other Non-cancerous Breast Conditions
- Breast Cancer Videos
- Breast Cancer Quiz
- Infographic: 7 Things to Know About Getting a Mammogram
- Frequently Asked Questions About the American Cancer Society’s Breast Cancer Screening Guideline
Researchers around the world are working to find better ways to prevent, detect, and treat breast cancer, and to improve the quality of life of patients and survivors.
Research studies
Breast cancer causes, breast cancer prevention, new tests to personalize your treatment, new imaging tests, breast cancer treatment, supportive care.
Current guidance on preventing and treating breast cancer as well as what might cause it (among other things) has come mainly from information discovered from research studies . Research studies can range from studies done in the lab to clinical trials done with hundreds of thousands of people. Clinical trials are carefully controlled studies that can gather specific information about certain diseases as well as explore promising new treatments.
Clinical trials are one way to get the latest cancer treatments that are being investigated. Still, they are not right for everyone. If you would like to learn more about clinical trials that might be right for you, start by asking your doctor if your clinic or hospital conducts clinical trials, or see Clinical Trials to learn more.
Studies continue to look at how certain lifestyle factors, habits, and other environmental factors, as well as inherited gene changes, might affect breast cancer risk. Here are a few examples:
- Several studies are looking at the effects of physical activity, weight gain or loss, and diet on breast cancer risk.
- Some breast cancers run in families, but many of the gene mutations (changes) that cause these breast cancers are not yet known. Research is being done to identify these gene changes.
- Several studies are focusing on the best use of genetic testing for inherited breast cancer gene mutations.
- Scientists are exploring how common gene variants (small changes in genes that are not as significant as mutations) may affect breast cancer risk. Gene variants typically have only a modest effect on risk by themselves, but when combined they could possibly have a large impact.
- Possible environmental causes of breast cancer have also received more attention in recent years. While much of the science on this topic is still in its earliest stages, this is an area of active research.
Researchers are looking for ways to help reduce breast cancer risk, especially for women who are at high risk. Here are some examples:
- Studies continue to look at whether certain levels of physical activity, losing weight, or eating certain foods, groups of foods, or types of diets might help lower breast cancer risk.
- Some hormonal medicines such as tamoxifen, raloxifene, exemestane, and anastrozole have already been shown to help lower breast cancer risk for certain women at higher risk. Researchers continue to study which groups of women might benefit most from these drugs.
- Clinical trials are also looking at whether some non-hormonal drugs might lower breast cancer risk, such as drugs used to treat blood or bone marrow disorders, like ruxolitinib.
- Studies are looking at vaccines that might help prevent certain types of breast cancer in people who are at high risk for breast cancer (due to presence of hereditary gene mutations or breast cancer in the family).
Breast cancer tissue is routinely tested for the biomarkers ER , PR , and HER2 to help make treatment decisions. A biomarker is any gene, protein, or other substance that can be measured in blood, tissues, or other body fluids. Some studies are looking at whether testing for other biomarkers, such as HER3, might also be helpful, but research on this is still in early phases.
Circulating tumor DNA (ctDNA) is DNA that is released into the bloodstream when cancer cells die. Identifying and testing the ctDNA in the blood for biomarkers is a rapidly growing area of study.
Some ways ctDNA might potentially be used in breast cancer include:
- Looking for new biomarkers in the tumor cells that might mean the cancer has become resistant to specific treatments (like chemo or targeted drug therapy)
- Determining if a certain drug will work on a tumor before trying it
- Predicting if the breast cancer will recur (come back) in women with early-stage breast cancer
- Predicting if neoadjuvant treatment is working to destroy the tumor instead of using imaging tests like a CT scan or US
- Determining if breast cancer or a high-risk breast condition is present before changes are found on an imaging test like a mammogram
Newer types of tests are being developed for breast imaging. Some of these are already being used in certain situations, while others are still being studied. It will take time to see if they are as good as or better than those used today. Some of these tests include:
- Scintimammography (molecular breast imaging)
- Positron emission mammography (PEM)
- Electrical impedance imaging (EIT)
- Elastography
- New types of optical imaging tests
For more on these tests, see Newer and Experimental Breast Imaging Tests .
New kinds of treatments for breast cancer are always being studied. For example, in recent years, several new targeted drugs have been approved to treat breast cancer.
But more and better treatment options are needed, especially for cancers like triple-negative breast cancer, where chemotherapy is the main option.
Some areas of research involving breast cancer treatment include:
- Studying if shorter courses of radiation therapy for very early-stage breast cancers are at least as good as the longer courses now often used
- Testing if different types of radiation therapy, such as proton beam radiation, might be better than standard radiation.
- Combining certain drugs (like 2 targeted drugs, a targeted drug with an immunotherapy drug, or a hormone drug with a targeted drug) to see if they work better together
- Trying to find new drugs or drug combinations that might help treat breast cancer that has spread to the brain
- Testing different immunotherapy drugs to treat triple-negative breast cancer
- Giving cancer vaccines to see if this helps keep the cancer from either worsening or coming back after treatment. There are many ways in which cancer vaccines work. For example, protein vaccines stimulate the immune system to recognize and attack specific cancer proteins. DNA vaccines contain DNA instructions so that once the vaccine is given, the DNA will instruct your body to make protein(s) to help the immune system recognize and attack cancer cells.
- Finding new ways to treat women with hereditary breast cancer, since they have a higher chance of the cancer recurring (coming back)
- Determining if chemotherapy is needed to treat every woman with HER2-positive breast cancer
- Finding new treatment options when breast cancer becomes resistant to current treatments
Supportive care helps patients and caregivers manage the symptoms of cancer and side effects of cancer treatment. Clinical trials are looking at different medicines and techniques to try to improve supportive care for people with breast cancer. For example, some studies are investigating:
- If there are better medicines or ways to prevent the damage to nerves that sometimes happen with certain chemotherapy drugs
- If drugs or other treatments might be helpful in limiting memory problems and other brain symptoms after chemotherapy
- If certain heart or blood pressure drugs, can help prevent the heart damage sometimes caused by common breast cancer drugs such as doxorubicin and trastuzumab
- If there are medicines that might be able to help treat the tired feeling that cancer can cause
Breast Cancer Research Highlights
The Society's research program has played a crucial role in saving lives from breast cancer. See examples of our current research.
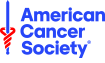
The American Cancer Society medical and editorial content team
Our team is made up of doctors and oncology certified nurses with deep knowledge of cancer care as well as editors and translators with extensive experience in medical writing.
Chan JCH, Chow JCH, Ho CHM, Tsui TYM, Cho WC. Clinical application of circulating tumor DNA in breast cancer. J Cancer Res Clin Oncol. 2021;147(5):1431-1442. doi:10.1007/s00432-021-03588-5.
Cullinane C, Fleming C, O’Leary DP, et al. Association of Circulating Tumor DNA With Disease-Free Survival in Breast Cancer: A Systematic Review and Meta-analysis. JAMA Netw Open. 2020;3(11):e2026921. doi:10.1001/jamanetworkopen.2020.26921.
Cuzick, J et al. Anastrozole for prevention of breast cancer in high-risk postmenopausal women (IBIS-II): an international, double-blind, randomised placebo-controlled trial. The Lancet. 2014;383 (9922):1041 - 1048.
Goss, P.E., et al., Exemestane for Breast-Cancer Prevention in Postmenopausal Women . New England Journal of Medicine , 2011. 364(25): p. 2381-2391.
Greene LR, Wilkinson D. The role of general nuclear medicine in breast cancer. J Med Radiat Sci . 2015;62(1):54-65.
Henry NL, Bedard PL, and DeMichele A. Standard and Genomic Tools for Decision Support in Breast Cancer Treatment. In Dizon DS, Pennel N, Rugo HS, Pickell LF, eds. 2017 American Society of Clinical Oncology Educational Book. 53 rd Annual Meeting. 2017.
Ignatiadis M, Lee M, and Jeffrey SS. Circulating Tumor Cells and Circulating Tumor DNA: Challenges and Opportunities on the Path to Clinical Utility. Clin Cancer Res ; 21(21); 4786–800.
Litton JK, Burstein HJ, Turner NC. Molecular Testing in Breast Cancer. Am Soc Clin Oncol Educ Book . 2019 Jan;39:e1-e7. doi: 10.1200/EDBK_237715. Epub 2019 May 17.
Magbanua MJM, Swigart LB, Wu HT, et al. Circulating tumor DNA in neoadjuvant-treated breast cancer reflects response and survival. Ann Oncol . 2021;32(2):229-239. doi:10.1016/j.annonc.2020.11.007.
Mayer IA, Dent R, Tan T, et al. Novel Targeted Agents and Immunotherapy in Breast Cancer. In Dizon DS, Pennel N, Rugo HS, Pickell LF, eds. 2017 American Society of Clinical Oncology Educational Book. 53 rd Annual Meeting. 2017.
National Cancer Institute. https://www.cancer.gov/about-cancer/treatment/clinical-trials/search. A Vaccine (Alpha-Lactalbumin) for the Treatment of Stage II-III Triple-Negative Breast Cancer. Accessed January 19, 2022.
National Cancer Institute. https://www.cancer.gov/about-cancer/treatment/clinical-trials/search. Bexarotene in Preventing Breast Cancer in Patients at High Risk for Breast Cancer. Accessed August 15, 2019.
National Cancer Institute. https://www.cancer.gov/about-cancer/treatment/clinical-trials/search. Donepezil Hydrochloride in Improving Memory Performance in Breast Cancer Survivors after Chemotherapy. Accessed August 15, 2019.
National Cancer Institute. https://www.cancer.gov/about-cancer/treatment/clinical-trials/search. Ruxolitinib in Preventing Breast Cancer in Patients with High Risk and Precancerous Breast Lesions. Accessed August 15, 2019.
National Cancer Institute. https://www.cancer.gov/about-cancer/treatment/clinical-trials/search. Testing the Addition of a Blood Pressure Medication, Carvedilol, to HER-2 Targeted Therapy for Metastatic Breast Cancer to Prevent Cardiac Toxicity. Accessed August 15, 2019.
National Institute of Environmental Health Sciences. Breast Cancer. Last reviewed November 15, 2021. Accessed January 19, 2022. https://www.niehs.nih.gov/health/topics/conditions/breast-cancer/index.cfm.
Rossi G, Mu Z, Rademaker AW, Austin LK, Strickland KS, Costa RLB et al. Cell-Free DNA and Circulating Tumor Cells: Comprehensive Liquid Biopsy Analysis in Advanced Breast Cancer. Clin Cancer Res. 2018 Feb 1;24(3):560-568.
Shoukry M, Broccard S, Kaplan J, Gabriel E. The Emerging Role of Circulating Tumor DNA in the Management of Breast Cancer. Cancers (Basel) . 2021;13(15):3813. Published 2021 Jul 29. doi:10.3390/cancers13153813.
Solin LJ, Gray R, Baehner FL, et al. A multigene expression assay to predict local recurrence risk for ductal carcinoma in situ of the breast. J Natl Cancer Inst . 2013;105:701-710.
Yu M, Bardia A, Aceto N et al. Ex vivo culture of circulating breast tumor cells for individualized testing of drug susceptibility. Science . 2014 Jul 11; 345(6193): 216–220.
Last Revised: February 15, 2024
American Cancer Society medical information is copyrighted material. For reprint requests, please see our Content Usage Policy .
American Cancer Society Emails
Sign up to stay up-to-date with news, valuable information, and ways to get involved with the American Cancer Society.
More in Breast Cancer
- About Breast Cancer
- Risk and Prevention
- Early Detection and Diagnosis
- Understanding a Breast Cancer Diagnosis
- Breast Reconstruction Surgery
- Living as a Breast Cancer Survivor
Help us end cancer as we know it, for everyone.

We fund research breakthroughs that save lives. Your year-end gift helps find new treatments for cancer.
Your Account
Manage your account, subscriptions and profile.
MyKomen Health
ShareForCures
In Your Community
In Your Community
View resources and events in your local community.
Change your location:
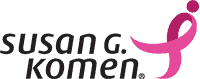
Susan G. Komen®
One moment can change everything.
What’s New in Breast Cancer
This section gives an overview of new breast cancer treatment breakthroughs and recent developments in research that are fueling new ways to assess risk, and prevent, detect, diagnose and treat breast cancer. Advances in breast cancer care are evaluated through a rigorous process that includes clinical trials and regulatory approvals before being considered standards of care and included in breast cancer care guidelines. Komen’s research team monitors the rapidly evolving breast cancer landscape, and here we will highlight new breast cancer treatment breakthroughs, innovations in technology or key advances that may be added or are new to guidelines. We will share these research advancements to empower patients with knowledge to help them make informed decisions with their doctors.
Use these links to jump to the topics below.
- Emerging Areas in Metastatic Breast Cancer Treatment
- Clinical Trials
Treatments and Drugs
For patients, new treatments can mean more options and more hope. Researchers are working to develop new breast cancer treatment breakthroughs, such as more effective drugs that will specifically target breast cancer cells, minimize side effects and prevent breast cancer cells from coming back. While some treatments increase the effectiveness of existing drugs, others may offer new, innovative strategies for attacking tumor cells.
As of August 2023, the following new treatments and drugs are currently in clinical trials and have not yet received FDA approval:
- A new antibody-drug conjugate called datopotamab deruxtecan (Dato-DXd) is currently being evaluated in three Phase 3 clinical trials for advanced estrogen receptor-positive (ER+) [1] breast cancer, metastatic triple negative [ 2 ] breast cancer and early triple negative [ 3 ] breast cancer (TNBC). Dato-DXd specifically targets a protein called TROP2, a biomarker that can be used to target cancer cells instead of healthy cells. Another TROP2-targeting therapy called sacituzumab govitecan has already been approved for TNBC and estrogen-receptor-positive breast cancer. Dato-DXd uses a different chemotherapy drug and delivery system compared to sacituzumab govitecan.
- People with metastatic estrogen receptor-positive breast cancer that progresses after their initial treatment are prone to developing mutations in the estrogen receptor (ER) gene (ESR1)[ 4 ]. ESR1 mutations cause the ER protein to be constantly active, driving tumor growth even in the presence of drugs designed to stop the ER from working. Lasofoxifene is a new type of hormone therapy being studied that stops the ER even when it’s mutated. Recent findings from the phase 2 ELAINE 2 clinical trial showed lasofoxifene plus the CDK4/6 inhibitor abemeciclib resulted in participants’ cancer remaining stable for a median of 13 months. Based on these results, the new phase 3 ELAINE 3 trial will compare lasofoxifene with the current standard of care fulvestrant (hormone therapy) in combination with a CDK4/6 inhibitor. If successful, patients may have a new hormone therapy option.
- Pembrolizumab is currently the only immunotherapy drug available for people with TNBC . The phase 2 BEGONIA clinical trial recently reported promising results for an immunotherapy drug called durvalumab (Imfinizi) in combination with a TROP-2 targeting antibody-drug conjugate being studied called Dato-DXd [ 5 ]. In this clinical trial, 62 people with metastatic triple-negative breast cancer were treated with this novel drug combination, and their cancer remained stable for a median of 13.8 months. Based on these exciting results, there are now three phase 3 clinical trials testing this drug combination in different breast cancer settings (TROPION-Breast03, TROPION-Breast04, TROPION-Breast05).
New and improved technologies may be able to increase the speed and accuracy of detecting, diagnosing or monitoring breast cancer for progression and response to treatment.
- Doctors may use PET scans, or positron emission tomography, to scan for evidence that breast cancer has spread or metastasized. Once breast cancer has spread, the metastases may have evolved to a different type of breast cancer than the original tumor. These differences mean the metastases and the original tumor may not respond to the same treatments. A diagnostic imaging agent called Cerianna (fluoroestradiol F-18 or FES PET) allows doctors to use PET scans to learn if estrogen receptors are present in metastatic lesions. If a person has metastatic lesions that are estrogen receptor-positive, they may respond well to hormone therapy. This agent was recently incorporated in the National Comprehensive Cancer Network (NCCN) guidelines [ 6 ] as an option for some people with metastatic or recurrent estrogen receptor-positive breast cancer to consider [ 7 ].
- Dormant cancer cells are cells that did not die from a person’s initial treatment. These cells can “hibernate” undetected for unknown reasons until they begin to grow again. The bone is a common place for dormant breast cancer cells to hide and possibly grow. In the phase 2 CLEVER clinical trial [ 8 ], presented at the European Society of Clinical Oncology in October 2023 by Komen Scholar Dr. Angela DeMichele, researchers tested whether they could find dormant cancer cells in participants’ bone marrow and eliminate them. Study results showed researchers were able to find and remove dormant cancer cells from about 80% of the participants. While larger studies will be needed to confirm these results, the CLEVER study shows this promising approach may prevent breast cancer recurrence .
- Doctors are getting closer to identifying which patients with early HER2-positive breast cancer can safely avoid chemotherapy by using the HER2DX genomic test. HER2DX is the first test specifically designed to identify HER2-positive patients at high and low risk for recurrence . For some people, being able to avoid chemotherapy without compromising long-term outcomes will lead to a better quality of life.
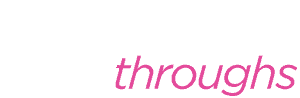
Research can take decades to reach the bedside, but what discoveries are just around the corner for patients? Susan G. Komen shares all of this and more through Breast Cancer Breakthroughs, a virtual education series focusing on the new science and technology advancements that are poised to make a difference for patients in the near future. Sign up for Breast Cancer Breakthroughs to never miss an episode.
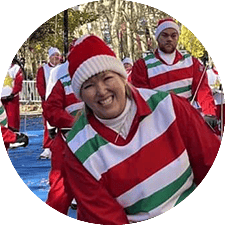
Kimberly’s Story: Finding Joy in the Midst of a Metastatic Breast Cancer Diagnosis
After Kimberly Reinika’s mother passed away in 2019 from ovarian cancer, she worried that it would ultimately take her life, too. “That was the cancer I was checking for,” she said.
Approaches to Care
With knowledge gained from clinical trials, researchers are seeking new ways to improve patient outcomes while using existing drugs. Some new breast cancer treatment breakthroughs are the result of combining certain drugs, finding which patients can skip certain elements of treatment or changing the order of their treatments to maximize effectiveness or minimize side effects.
- All people with early TNBC are currently treated with immunotherapy and chemotherapy prior to surgery , then they receive immunotherapy for up to 27 weeks after their surgery. A new phase 3 clinical trial called OptimICE-PCR led by Komen Scholar Dr. Sara Tolaney, will test a new approach. The study will determine whether simply observing patients is as effective as getting immunotherapy after surgery in preventing breast cancer from coming back, if their initial treatment successfully got rid of all the breast cancer [ 9 ]. While effective, immunotherapy comes with side effects and may not be needed in some treatment plans. This study will help identify who can safely de-escalate their immunotherapy treatment while maintaining good outcomes.
- Results from the phase 3 DESTINY-Breast06 clinical trial [ 10 ], presented at the American Society of Clinical Oncology (ASCO) meeting in June 2024, showed patients with metastatic estrogen receptor-positive (ER-positive), HER2-low and HER2-ultralow breast cancer had about a 5-month progression-free survival benefit with trastuzumab deruxtecan compared to chemotherapy . HER2-ultralow is a new designation and means that there is a very small amount of detectable HER2 in a tumor. Trastuzumab deruxtecan has already been shown to be effective in HER2-low metastatic breast cancer, but this is the first study showing that people with HER2-ultralow metastatic breast cancer may benefit as well. With these new findings, about 85% of patients with metastatic ER-positive breast cancer may become eligible for this treatment.
- New data from the Young Women’s Breast Cancer Study, led by Komen Chief Scientific Advisor Dr. Ann Partridge, found 73% of women with stage I-III breast cancer who attempted to get pregnant after completing their breast cancer treatment were successful. [ 11 ] This study, presented at the 2024 Annual ASCO meeting, is one of the most comprehensive studies attempting to answer this question to date. The results highlight the importance of making sure women have access to fertility preservation when they begin their breast cancer treatment.
- Komen Scholar Dr. Bryan Schneider conducted the phase 2 EAZ171 clinical trial, which tested whether certain gene mutations could predict the likelihood of developing a side effect from some types of chemotherapy called taxane-induced peripheral neuropathy (TIPN), which is more common among Black women. This side effect causes pain, numbness and tingling in the extremities and can also lead to the treatment being stopped. Komen grantee Dr. Tarah Ballinger presented the results of the EAZ171 study at the 2024 Annual ASCO meeting. [ 12 ] The study found that while the gene mutations were unable to predict the likelihood of developing TIPN, researchers did identify a chemotherapy regimen that resulted in fewer instances of TIPN. These results provide some of the best evidence available to date to personalize chemotherapy treatment for Black women .
Komen will be closely monitoring the results of these studies and more at upcoming scientific conferences and hopes to see more promising data regarding new ways to prevent, detect, diagnose and treat breast cancer.
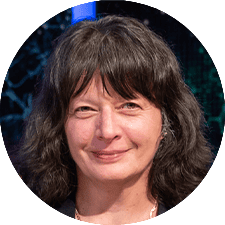
It Looks Promising: Uncovering New Possibilities in Breast Cancer Prevention
Is breast cancer prevention possible? Komen Scientific Advisory Board Member Dr. Kornelia Polyak is exploring a new strategy to identify and eliminate cell precursors from which tumors can grow.

Help discover cures to breast cancer, faster. New treatment breakthroughs for breast cancer come from researchers learning from people who have breast cancer, but our current data sources only represent a small portion of the breast cancer community. Help us discover the cures to breast cancer, faster, by joining ShareForCures.
What’s New in Breast Cancer References
- https://classic.clinicaltrials.gov/ct2/show/NCT05104866
- https://clinicaltrials.gov/study/NCT05374512
- https://classic.clinicaltrials.gov/ct2/show/NCT05629585
- https://breast-cancer-research.biomedcentral.com/articles/10.1186/s13058-021-01462-3
- https://oncologypro.esmo.org/meeting-resources/esmo-congress/datopotamab-deruxtecan-dato-dxd-durvalumab-d-as-first-line-1l-treatment-for-unresectable-locally-advanced-metastatic-triple-negative-breast
- https://www.gehealthcare.com/about/newsroom/press-releases/ge-healthcare-announces-fes-pet-imaging-recommendation-in-nccn-clinical-practice-guidelines-in-oncology-nccn-guidelines
- https://www.nccn.org/patients/guidelines/content/PDF/breast-invasive-patient.pdf (page 16)
- https://ascopost.com/news/october-2023/novel-strategies-for-eliminating-dormant-tumor-cells-in-breast-cancer-survivors/
- https://www.cancer.gov/research/participate/clinical-trials-search/v?id=NCI-2022-07859&r=1
- https://ascopost.com/news/june-2024/t-dxd-improves-progression-free-survival-in-patients-with-breast-cancer-previously-treated-with-endocrine-therapy/
- https://www.dana-farber.org/newsroom/news-releases/2024/most-young-women-treated-for-breast-cancer-can-have-children-study-shows#:~:text=Most%20young%20women%20treated%20for%20breast%20cancer%20can%20have%20children%2C%20study%20shows,-Posted%20date&text=New%20research%20by%20Dana%2DFarber,and%20want%20to%20have%20children.
- https://www.komen.org/blog/personalized-chemo/
TOOLS & RESOURCES

NEED HELP OR MORE INFORMATION?
1-877 GO KOMEN (1-877-465-6636)

Educational Resources
Komen Financial Assistance Program
Breast Cancer Clinical Trials
Clinical trials are research studies that involve people. The clinical trials on this list are for breast cancer. All trials on the list are NCI-supported clinical trials, which are sponsored or otherwise financially supported by NCI.
NCI’s basic information about clinical trials explains the types and phases of trials and how they are carried out. Clinical trials look at new ways to prevent, detect, or treat disease. You may want to think about taking part in a clinical trial. Talk to your doctor for help in deciding if one is right for you.
This phase III trial compares the addition of chemotherapy to usual treatment (ovarian function suppression plus hormonal therapy) to usual treatment alone in treating premenopausal estrogen receptor (ER)-positive/HER2-negative breast cancer patients who are at high risk of their cancer returning. One of the likely benefits of chemotherapy is that it can stop the ovaries from releasing eggs and hormones in women who are premenopausal. This study may help researchers determine if adding chemotherapy to ovarian suppression and hormonal therapy has similar effectiveness to ovarian suppression and hormonal therapy alone in preventing cancer from returning.
This phase III trial compares the effect of usual treatment with trastuzumab emtansine (T-DM1) alone vs. T-DM1 in combination with tucatinib. T-DM1 is a monoclonal antibody, called trastuzumab, linked to a chemotherapy drug, called DM1. Trastuzumab is a form of targeted therapy because it attaches to specific molecules (receptors) on the surface of cancer cells, known as HER2 receptors, and delivers DM1 to kill them. Tucatinib blocks HER2, which may help keep cancer cells from growing and may kill them. Giving T-DM1 in combination with tucatinib may work better in preventing breast cancer from relapsing in patients with HER2 positive breast cancer compared to T-DM1 alone.
This phase III trial compares the effects of chemotherapy immunotherapy (chemo-immunotherapy) that is both shorter and does not include anthracyclines to usual chemo-immunotherapy for the treatment of early triple negative breast cancer. Paclitaxel is a taxane and in a class of medications called anti-microtubule agents. It stops cancer cells from growing and dividing and may kill them. Carboplatin is in a class of medications known as platinum-containing compounds. It works in a way similar to the anticancer drug cisplatin, but may be better tolerated than cisplatin. Carboplatin works by killing, stopping or slowing the growth of cancer cells. Cyclophosphamide is in a class of medications called alkylating agents. It works by damaging the cell’s deoxyribonucleic acid (DNA) and may kill cancer cells. It may also lower the body’s immune response. Docetaxel is in a class of medications called taxanes. It stops cancer cells from growing and dividing and may kill them. Doxorubicin is in a class of medications called anthracyclines. Doxorubicin damages the cell’s DNA and may kill cancer cells. It also blocks a certain enzyme needed for cell division and DNA repair. Pembrolizumab may stop the growth of tumor cells by blocking some of the enzymes needed for cell growth. Shorter chemo-immunotherapy treatment that uses fewer drugs (without anthracyclines) may be similar to the usual treatment (with anthracyclines) for triple negative breast cancer.
This phase III trial compares the effect of radiation therapy combined with hormonal therapy versus hormonal therapy alone in treating patients with low risk, early stage breast cancer with Oncotype Dx Recurrence =< 18. Oncotype DX is a laboratory test which results in a score that is used to help predict whether breast cancer will spread to other parts of the body or come back. Radiation therapy uses high doses of radiation to kill cancer cells and shrink tumors but may result in some side effects. Hormones called estrogen and progesterone may contribute to the growth of breast tumor cells. Hormone therapy, also called endocrine therapy, may stop the growth of tumor cells by blocking or removing these hormones. This clinical trial may help researchers understand if patients with low-risk, early stage breast cancer who have Oncotype recurrence score of =< 18 can safely omit radiation therapy and only be treated with hormonal therapy without losing any radiation treatment benefit.
This phase III trial compares the effect of continuation of treatment with pembrolizumab (usual approach) to observation only at preventing cancer from coming back in patients with early-stage triple-negative breast cancer (TNBC) who achieved a pathologic complete response after preoperative chemotherapy in combination with pembrolizumab. The usual approach for patients with early-stage TNBC who receive preoperative chemotherapy plus pembrolizumab is to continue to receive pembrolizumab for up to 27 weeks after surgery. Immunotherapy with monoclonal antibodies, such as pembrolizumab, may help the body's immune system attack the cancer, and may interfere with the ability of tumor cells to grow and spread. This trial may help researchers determine if observation is as good as receiving pembrolizumab for 27 weeks after surgery in triple-negative breast cancer patients who achieved a pathologic complete response after preoperative treatment with chemotherapy and pembrolizumab.
This randomized phase III trial studies if not giving regional radiotherapy is just as good as using regional radiotherapy in keeping breast cancer from coming back in patients with estrogen receptor (ER) positive, HER2 negative node positive low risk breast cancer who have undergone breast conserving surgery or mastectomy. Women with ER positive breast cancer normally will receive endocrine therapy and some may receive chemotherapy to help prevent the cancer from coming back. Many women will also receive radiotherapy to the whole breast/chest area and the surrounding lymph glands (called regional radiotherapy). Radiation therapy uses high energy x-rays to kill tumor cells and shrink tumors. It is not known whether patients with low risk breast cancer need to receive regional radiotherapy. As a result, some women may be getting regional radiotherapy who do not need it and be exposed to the side effects of their treatment without benefit. This study will help to determine if regional radiotherapy can be omitted for low risk ER positive node positive breast cancer patients.
This phase III trial compares the addition of an immunotherapy drug (durvalumab) to usual chemotherapy versus usual chemotherapy alone in treating patients with MammaPrint Ultrahigh (MP2) stage II-III hormone receptor positive, HER2 negative breast cancer. Immunotherapy with monoclonal antibodies, such as durvalumab, may help the body's immune system attack the cancer, and may interfere with the ability of tumor cells to grow and spread. Chemotherapy drugs, such as paclitaxel, doxorubicin, and cyclophosphamide work in different ways to stop the growth of tumor cells, either by killing the cells, by stopping them from dividing, or by stopping them from spreading. There is some evidence from previous clinical trials that people who have a MammaPrint Ultrahigh Risk result may be more likely to respond to chemotherapy and immunotherapy. Adding durvalumab to usual chemotherapy may be able to prevent the cancer from returning for patients with MP2 stage II-III hormone receptor positive, HER2 negative breast cancer.
This phase III trial compares the usual treatment of breast radiation and HER2-targeted drugs following surgery and chemotherapy to using the usual treatment approach without radiation in treating patients with low-risk HER2-positive breast cancer. The usual approach is defined as care most people get for this type of cancer. The usual approach for patients who have undergone surgery, chemotherapy, and HER2-targeted therapy and are not in a study is treatment with radiation therapy to the breast and continuing HER2-targeted therapy. HER2-targeted drugs attach to the HER2 receptor proteins on the surface of breast tumor cells and block the HER2 receptors from receiving growth signals in HER2-positive breast cancer. By blocking the growth signals, HER2-targeted drugs can slow or stop the growth of HER2-positive breast cancer. Radiation therapy uses high energy sources of radiation to kill tumor cells and shrink tumors. The usual approach for patients who have undergone surgery, chemotherapy, and HER2-targeted therapy and are not in a study is treatment with radiation therapy to the breast and continuing HER2-targeted therapy. Omitting radiation from the usual treatment approach may avoid the potential short-term and long-term risks of radiation in patients with low-risk HER2-positive breast cancer and may have the same effect as the usual approach in preventing cancer from coming back (recurrence ).
This phase II ComboMATCH treatment trial compares the usual treatment alone (fulvestrant) to using binimetinib plus the usual treatment in patients with hormone receptor positive breast cancer that has spread from where it first started to other places in the body (metastatic) and has an NF1 genetic change. Fulvestrant is a hormonal therapy that binds to estrogen receptors in tumor cells, resulting in estrogen receptor destruction and decreased estrogen binding, which may inhibit the growth of estrogen-sensitive tumor cells. Binimetinib is a targeted therapy that may stop the growth of tumor cells by blocking some of the enzymes needed for cell growth. The addition of binimetinib to fulvestrant in breast cancers with an NF1 genetic change could increase the percentage of tumors that shrink as well as lengthen the time that the tumors remain stable (without progression) as compared to fulvestrant alone.
This phase II trial studies the effect of sacituzumab govitecan in treating patients with HER2-negative breast cancer that has spread to the brain (brain metastases). Sacituzumab govitecan is a monoclonal antibody, called sacituzumab, linked to a chemotherapy drug, called govitecan. Sacituzumab is a form of targeted therapy because it attaches to specific molecules on the surface of cancer cells, known as Trop-2 receptors, and delivers govitecan to kill them. Giving sacituzumab govitecan may shrink the cancer in the brain and/or extend the time until the cancer gets worse.
This phase III trial compares the effectiveness of fractionated stereotactic radiosurgery (FSRS) to usual care stereotactic radiosurgery (SRS) in treating patients with cancer that has spread from where it first started to the brain. Radiation therapy uses high energy x-rays to kill tumor cells and shrink tumors. FSRS delivers a high dose of radiation to the tumor over 3 treatments. SRS is a type of external radiation therapy that uses special equipment to position the patient and precisely give a single large dose of radiation to a tumor. FSRS may be more effective compared to SRS in treating patients with cancer that has spread to the brain.
The main purpose of this study is to measure how well imlunestrant works compared to standard hormone therapy in participants with early breast cancer that is estrogen receptor positive (ER+) and human epidermal receptor 2 negative (HER2-). Participants must have already taken endocrine therapy for two to five years and must have a higher-than-average risk for their cancer to return. Study participation could last up to 10 years.
The goal of this study is to find out if the experimental product, sacituzumab govitecan-hziy (SG) in combination with pembrolizumab given after surgery, is effective and safe compared to the treatment of physician's choice (TPC) which includes either pembrolizumab or pembrolizumab plus capecitabine in participants with triple negative breast cancer that still remains after surgery and pre-surgical treatment.
This is a Phase III, randomized, open-label, 3-arm, multicenter, international study assessing the efficacy and safety of Dato-DXd with or without durvalumab compared with ICT in participants with stage I to III TNBC with residual invasive disease in the breast and/or axillary lymph nodes at surgical resection following neoadjuvant systemic therapy.
A randomized, Phase II trial of circulating tumor DNA-guided second line Adjuvant therapy for high Residual risk, stage II-III, Estrogen Receptor positive, HER-2 negative breast cancer (DARE)
The purpose of this study is to further advance the ability to practice personalized medicine by learning which new drug agents are most effective with which types of breast cancer tumors and by learning more about which early indicators of response (tumor analysis prior to surgery via magnetic resonance imaging (MRI) images along with tissue and blood samples) are predictors of treatment success.
This phase II trial studies the effects of trastuzumab emtansine followed by trastuzumab versus paclitaxel with trastuzumab in treating patients with HER2-positive stage I breast cancer. Trastuzumab emtansine is a monoclonal antibody, called trastuzumab, linked to a chemotherapy drug called emtansine. Trastuzumab attaches to HER2 positive cancer cells in a targeted way and delivers emtansine to kill them. Chemotherapy drugs, such as paclitaxel, work in different ways to stop the growth of tumor cells, either by killing the cells, by stopping them from dividing, or by stopping them from spreading. Trastuzumab is a monoclonal antibody, which are disease-fighting proteins made by cloned immune cells. Giving trastuzumab emtansine followed by trastuzumab may have less side-effects than traditional HER2-positive breast cancer treatment of trastuzumab and paclitaxel.
This phase II trial studies the effect of hormonal therapy given after (adjuvant) combination pertuzumab/trastuzumab in treating patients with hormone receptor positive, HER2 positive breast cancer. The drugs trastuzumab and pertuzumab are both monoclonal antibodies, which are disease-fighting proteins made by cloned immune cells. Estrogen can cause the growth of breast cancer cells. Hormonal therapy, such as letrozole, anastrozole, exemestane, and tamoxifen, block the use of estrogen by the tumor cells. Giving hormonal therapy after pertuzumab and trastuzumab may kill any remaining tumor cells in patients with breast cancer.
This is a Phase III, 2-arm, randomised, open-label, multicentre, global study assessing the efficacy and safety of neoadjuvant Dato-DXd plus durvalumab followed by adjuvant durvalumab with or without chemotherapy compared with neoadjuvant pembrolizumab plus chemotherapy followed by adjuvant pembrolizumab with or without chemotherapy in participants with previously untreated TNBC or hormone receptor-low/HER2-negative breast cancer.
This is a Phase 3, open-label, randomized, clinical trial evaluating the efficacy and safety of gedatolisib plus fulvestrant with or without palbociclib for the treatment of patients with locally advanced or metastatic HR+/HER2- breast cancer following progression on or after CDK4/6 and aromatase inhibitor therapy.
This phase II trial studies how well nab-paclitaxel, durvalumab, and tremelimumab with or without personalized synthetic long peptide vaccine (neoantigen vaccine) works in treating patients with triple negative breast cancer that has spread from where it first started (primary site) to other places in the body (metastatic). Chemotherapy drugs, such as nab-paclitaxel, work in different ways to stop the growth of tumor cells, either by killing the cells, by stopping them from dividing, or by stopping them from spreading. Immunotherapy with monoclonal antibodies, such as durvalumab and tremelimumab, may induce changes in body’s immune system and may interfere with the ability of tumor cells to grow and spread. Vaccines made from peptides may help the body build an effective immune response to kill tumor cells. It is not yet known whether giving nab-paclitaxel, durvalumab, and tremelimumab with or without neoantigen vaccine will work better in treating patients with triple negative breast cancer.
This is a Phase III open-label study to assess if camizestrant improves outcomes compared to standard endocrine therapy in patients with ER+/HER2 - early breast cancer with intermediate or high risk for disease recurrence who completed definitive locoregional therapy (with or without chemotherapy) and standard adjuvant endocrine therapy (ET) for at least 2 years and up to 5 years. The planned duration of treatment in either arm of the study is 60 months.
The goal of this clinical trial is to assess the efficacy, safety and tolerability of the combination of lasofoxifene and abemaciclib compared to fulvestrant and abemaciclib for the treatment of pre- and postmenopausal women and men who have previously received ribociclib or palbociclib-based treatment and have locally advanced or metastatic estrogen receptor positive (ER+)/human epidermal growth factor 2 negative (HER2-) breast cancer with an estrogen receptor 1 (ESR1) mutation. The main question the study aims to answer is: • To compare the efficacy of the combination of lasofoxifene and abemaciclib with that of fulvestrant and abemaciclib Participants will receive either receive 5 mg/d of oral lasofoxifene plus oral abemaciclib 150 mg twice a day or the combination of fulvestrant 500 mg intramuscular (IM) on Days 1, 15, and 29 and then once monthly thereafter plus oral abemaciclib 150 mg twice a day.
This is a Phase III open-label study to assess if camizestrant improves outcomes compared to standard adjuvant endocrine therapy for patients with ER+/HER2- early breast cancer with intermediate-high or high risk for disease recurrence who completed definitive locoregional therapy (with or without chemotherapy). The planned duration of treatment in either arm within the study will be 7 years.
A Phase Ib/III Open-label, Randomised Study of Capivasertib plus CDK4/6 Inhibitors and Fulvestrant versus CDK4/6 Inhibitors and Fulvestrant in Hormone Receptor-Positive and Human Epidermal Growth Factor Receptor 2-Negative Locally Advanced, Unresectable or Metastatic Breast Cancer (CAPItello-292)

An official website of the United States government
The .gov means it's official. Federal government websites often end in .gov or .mil. Before sharing sensitive information, make sure you're on a federal government site.
The site is secure. The https:// ensures that you are connecting to the official website and that any information you provide is encrypted and transmitted securely.
- Publications
- Account settings
- Browse Titles
NCBI Bookshelf. A service of the National Library of Medicine, National Institutes of Health.
Wild CP, Weiderpass E, Stewart BW, editors. World Cancer Report: Cancer research for cancer prevention. Lyon (FR): International Agency for Research on Cancer; 2020.

World Cancer Report: Cancer research for cancer prevention.
5.9 breast cancer.
Susan E. Hankinson , Kornelia Polyak , Judy E. Garber , and Benjamin O. Anderson , reviewer. Valerie McCormack , reviewer.
Exposures occurring in utero and until menopause can influence breast cancer risk. Therefore, prevention efforts should be considered throughout a woman’s life.
Some breast cancer risk factors (e.g. mammographic density) are similarly associated with most currently recognized breast cancer subtypes, whereas for others (e.g. parity) the relationships vary significantly by subtype; reliable estimates of these differences have only recently begun to emerge.
Tumour subtypes should be considered when evaluating etiology and in developing prevention strategies.
Breast cancer risk conferred by an increasing number of high-penetrance predisposition genes has been better quantified and characterized. Panels of single-nucleotide polymorphisms both modify penetrance of the strong susceptibility genes and confer quantifiable breast cancer risk themselves.
Large population studies and major international collaborations, particularly those integrating new technologies and basic science discoveries, are providing novel insights into breast cancer etiology and prevention.
Emerging data indicate that many risk factors directly influence the numbers and/or properties of breast epithelial progenitors.
Fundamentals.
Breast cancer is a heterogeneous disease, with wide variation in tumour morphology, molecular characteristics, and clinical response. Invasive ductal carcinoma is the most common type of breast cancer, making up about 70% of tumours, and about 15–20% of tumours are invasive lobular carcinomas.
Assessment of the estrogen receptor (ER), progesterone receptor (PR), and human epidermal growth factor receptor 2 (HER2) expression status of tumours has been used in clinical decision-making for many years. Tumour molecular subtypes have subsequently been identified, for example on the basis of prognostic multigene classifiers, to derive at least the luminal A, luminal B, HER2-enriched, and basal-like classifications.
The importance of distinguishing between ER-positive and ER-negative breast cancer in epidemiological studies of etiology and prevention is now established. Studies linking risk factors with specific molecular subtypes of breast cancer are more recent, and several consistent findings, noted below, have emerged. Most recently, several subtypes of triple-negative (i.e. ER-negative, PR-negative, and HER2-negative) breast cancer have been identified [ 1 ], but these have yet to be considered in epidemiological studies.
- Epidemiology
Breast cancer is the most commonly diagnosed cancer type and the leading cause of cancer death in women worldwide. In 2018, there were an estimated 2.1 million new cases of breast cancer and 627 000 deaths from breast cancer worldwide [ 2 ]. The incidence and mortality rates show marked international variation ( Fig. 5.9.1 and Fig. 5.9.2 ). However, incidence and mortality data remain extremely limited for several world regions, such as Africa.
Fig. 5.9.1
Global distribution of estimated age-standardized (World) incidence rates (ASR) per 100 000 person-years for breast cancer in women, 2018.
Fig. 5.9.2
Global distribution of estimated age-standardized (World) mortality rates (ASR) per 100 000 person-years for breast cancer in women, 2018.
More than half of breast cancer cases are now diagnosed in low- and middle-income countries [ 3 ], where a greater proportion of cases (and sometimes a markedly greater proportion) are diagnosed at later stages, which are linked to poorer survival (see Chapter 1.3) ( Fig. 5.9.3 ). Continuing reductions in the prevalence of infectious diseases and associated increases in life expectancy, along with changes in population reproductive patterns (e.g. later age at first birth) and lifestyle factors (e.g. increasing obesity) portend an ever-increasing burden of breast cancer in low- and middle-income countries [ 3 ].
Fig. 5.9.3
Percentage of breast cancer cases diagnosed at a late stage (stages III and IV combined), by country or region and by time period or population group.
- Genetics and genomics
An inherited component to breast cancer susceptibility has long been recognized. Progress in recent years has included the identification of multiple breast cancer susceptibility genes, improved estimates of their penetrance, the identification of modifier genes, and increases in the yield of genome-wide association studies (GWAS) for breast cancer both overall (i.e. all subtypes of breast cancer combined) and by subtype [ 4 ].

High-penetrance gene mutations
The most common high-penetrance susceptibility alleles remain BRCA1 and BRCA2 , both of which are critical for repair of DNA double-strand breaks and remodelling of stalled replication forks. Data and specimens from large cohorts of well-characterized germline mutation carriers, such as the Consortium of Investigators of Modifiers of BRCA1/2 (CIMBA), have permitted stable estimates of breast cancer risk [5].
Other genes involved in DNA repair (see Chapter 3.4) were identified through mechanistic studies elucidating DNA repair pathways, Fanconi anaemia complementation groups, and interacting genes associated with novel functions of known genes [ 4 ,6]. The widespread adoption of next-generation sequencing technologies has led to the identification of germline mutations in individuals and families without classic phenotypic characteristics of a syndrome or syndromes associated with specific gene mutations, suggesting important selection bias in early studies (e.g. TP53 , CDH1 ) [6,7].
BRCA1 and BRCA2 have been studied in the greatest detail in large collaborative cohorts (e.g. CIMBA), from which the available data include genotype–phenotype correlations and the identification of modifier single-nucleotide polymorphisms (SNPs) [8], although none are yet used clinically to improve individual risk prediction. Examination of somatic and germline mutational signatures ( Fig. 5.9.4 ) may provide clues to breast cancer etiology based on specific patterns of acquired DNA alteration [9].
Fig. 5.9.4
Pathway enrichment map for susceptibility loci based on summary association statistics for 65 new breast cancer loci. Each coloured circle (node) represents a pathway (gene set), coloured by enrichment score, where redder nodes indicate lower false discovery (more...)
Susceptibility loci
Recent GWAS analyses (see Chapter 3.2) have increased in size [10] and have yielded multiple new susceptibility loci both for breast cancer overall and for specific breast cancer subtypes, especially triple-negative breast cancer [11]. A group of SNPs has been included in a personalized risk score that shows increased risk of breast cancer in women with and without a family history of breast cancer [12]. One cluster of SNPs has been shown to improve the performance of the Tyrer–Cuzick breast cancer risk prediction model, with the incorporation of mammographic density as well. These loci are entering clinical use, but most have been subjected to only limited validation [13].
Several reproductive and lifestyle factors are confirmed contributors to breast cancer risk. In recent years, the understanding of the impact of these exposures on risk has been improved largely through assessment of these exposures over a woman’s lifetime, according to breast tumour subtype, and through detailed assessments in large consortia.
Lifestyle and environmental exposures
A notable aspect of breast cancer etiology is the long-term influence of exposures experienced over the life-course. The best current example is body size (see Chapter 2.7): birth weight is positively associated with breast cancer risk; childhood, adolescent, and premenopausal body size are inversely related to risk; and postmenopausal body size is positively related to risk [14]. On the basis of recent data from 19 prospective cohorts, the inverse association with larger adult body size in premenopausal women is strong and linear [15] and is apparent for both ER-positive and ER-negative disease and across race and ethnicity [15]; furthermore, on the basis of a large Mendelian randomization study [16], the association is probably causal. Multiple studies also have assessed childhood and adolescent body size and have noted similar inverse associations [14]. Mechanistic understanding of these inverse associations may offer future targets for prevention.
A consortium analysis with more than 36 000 breast cancer cases reported that long duration of smoking before a first pregnancy was associated with a significant 18% (95% confidence interval [CI], 12–24%) increase in breast cancer risk; the associations were not confounded by current alcohol consumption and were observed predominantly for ER-positive tumours [17]. These data support a causal link of smoking with breast cancer risk and re-emphasize the importance of smoking prevention and cessation programmes in adolescents and young adults (see “Tobacco cessation: the WHO perspective”).
Studies suggest that carotenoids, or other constituents in carotenoid-rich foods, may decrease breast cancer risk [14], particularly for ER-negative disease; similarly, several studies have observed an inverse association between a Mediterranean diet score and ER-negative breast cancer [18].
The potential role of environmental and occupational exposures in breast carcinogenesis has remained a major interest, although challenges in exposure assessment and study design have limited the conclusions. Increasingly, efforts have focused on evaluating exposure during windows of susceptibility, by assessing links between contaminants and intermediate markers of risk such as breast density, and by increasing transdisciplinary research efforts. Such efforts are providing new insights into the potential for exposures such as endocrine disrupters to influence breast cancer risk [19].
Reproductive factors
The inverse association observed between parity and risk of breast cancer overall is consistently seen for ER-positive disease, whereas no association or a positive association has been observed for ER-negative and triple-negative disease [20]. In addition, breastfeeding has been associated with lower risk of hormone receptor-negative (including ER-negative, triple-negative, and basal-like) breast cancer; weaker and less consistent associations have been observed for ER-positive tumour subtypes [21]. These studies have been conducted largely in populations of European ancestry. Recently, across four studies of African American women, parity was observed to significantly increase risk of ER-negative and triple-negative breast cancer, and to modestly lower risk of ER-positive breast cancer. Women who breastfed versus never breastfed had lower risk of ER-negative and triple-negative disease ( Fig. 5.9.5 ) [22]. Importantly from a prevention perspective, breastfeeding appears to reduce risk of these breast cancer subtypes that have poorer prognosis. (For a discussion of reproductive factors such as age at menarche, age at first birth, and age at menopause, see Chapter 3.6.)
Fig. 5.9.5
Relative risks (with 95% confidence intervals) for number of births in relation to estrogen receptor (ER) status, according to history of breastfeeding, from the African American Breast Cancer Epidemiology and Risk (AMBER) Consortium. The reference for (more...)
Breast tumour subtypes
Studies have increasingly focused on evaluating risk factors by molecular characteristics of breast tumours, to provide causal insight for observed associations and to better inform prevention strategies. The differential associations of postmenopausal obesity and use of hormone therapy with ER-positive but not ER-negative breast cancer are established; differences observed more recently include dietary factors [14,18]. Furthermore, associations of parity and breastfeeding with risk appear to vary by molecular subtype [20,22,23]. Such analyses require both large sample sizes and the availability of tumour tissue; hence, reliable estimates of these differences have only recently begun to emerge.
Population attributable risks
Several recent efforts have evaluated the population attributable risks for breast cancer. In a study that combined data from two large cohorts and assessed a range of well-established breast cancer risk factors in relation to breast cancer in postmenopausal women, the population attributable risk was 70.0% (95% CI, 55.0–80.7%) overall [24]. For modifiable risk factors only, the population attributable risk was 34.6% overall and was higher for ER-positive tumours (39.7%) than for ER-negative tumours (27.9%) [24].
The Breast Cancer Surveillance Consortium reported that 52.7% and 54.7% of breast cancers in premenopausal and postmenopausal women, respectively, could potentially be attributed to six risk factors: Breast Imaging Reporting and Data System (BI-RADS) breast density, parity, age at first birth, body mass index (BMI), first-degree family history of breast cancer, and personal history of benign breast disease; the greatest contributors to these estimates were BMI and breast density [25].
- Biological characteristics
Endogenous hormones
Substantial progress has been made to further the understanding of the link between endogenous hormone concentrations, measured in blood or urine, and risk of breast cancer. Postmenopausal levels of estradiol and testosterone are established risk factors, with relative risks of breast cancer of 1.5–3.0 when comparing women in the top versus the bottom 20–25% of hormone levels.
Data in premenopausal women have been sparse, largely because of complexities in measuring estrogen levels during the menstrual cycle. In a recent pooled analysis of prospective studies with 767 cases and 1699 controls, a modest but significant positive association was noted for estradiol and testosterone levels in premenopausal women, with comparable relative risks of 1.41 ( P trend = 0.01) for estradiol and 1.32 ( P trend = 0.02) for testosterone ( Fig. 5.9.6 ); no association was observed for plasma progesterone levels [26]. A positive association between prolactin levels and risk of breast cancer, primarily in postmenopausal women, also has increasingly been documented [27].
Fig. 5.9.6
Relative risks (with 95% confidence intervals) of premenopausal breast cancer by quantile of circulating hormone concentrations.
Estrogen metabolites have been hypothesized to independently influence risk via effects on proliferation or by inducing oxidative damage. With an improved assay technology [28] used across five studies in postmenopausal women, a relative increase in levels of 2-hydroxylation pathway metabolites versus 16-hydroxylation pathway metabolites was associated with a 34% decrease (95% CI, 16–48%) in breast cancer risk independent of total estrogen levels [29]. Data in premenopausal women are limited but are suggestive of similar associations [28].
Anti-Müllerian hormone is produced by the ovaries, is measurable only before menopause, reflects the size of the ovarian follicular pool, and is strongly correlated with age at menopause [30]. In a large consortium analysis of 10 prospective studies, a significant positive association was observed, with a multivariable relative risk comparing the top versus the bottom quartile categories of 1.60 (95% CI, 1.31–1.94; P trend < 0.001) ( Fig. 5.9.6 ) [30]. The findings were unchanged after accounting for testosterone concentrations, were similar regardless of menopausal status at diagnosis, and were observed primarily for ER-positive tumours. Anti-Müllerian hormone is one of the few hormones assessed in premenopausal women that is now confirmed to predict later risk of breast cancer. Additional facets of this association, as well as the biological mechanisms underlying the association, require further study.
Novel technologies
New analytical technologies such as metabolomics and proteomics (see Chapter 3.7) can be used in population-based studies and are beginning to provide new insights into the biological mechanisms underlying known breast cancer risk factors, as well as offering the potential to identify new biomarkers of risk or early detection. For example, several diet-related metabolites (related to alcohol, vitamin E, and animal fat) were associated with risk of breast cancer, particularly for ER-positive disease, thus suggesting additional factors that may play a mechanistic role underlying these dietary exposures and modulation of risk [31].
Risk stratification
Breast cancer risk prediction models have been developed to estimate the risk of carrying a high-risk germline mutation, the risk of developing breast cancer, or both [32]. Until recently, existing models, such as the Breast Cancer Risk Assessment Tool (also known as the Gail model) and the Rosner–Colditz model, generally included reproductive factors, family history of breast cancer, and a subset of lifestyle factors. Recent work has suggested significant improvements in model performance with the addition of several biological markers, including mammographic breast density, genetic risk scores, and plasma endogenous hormone levels (e.g. [12,33]). Further enhancements are needed, including incorporation of newly confirmed risk factors (e.g. anti-Müllerian hormone), more specific disease definitions, and development and validation in a wider range of study populations. Other priorities are assessment of clinical utility and strategies to successfully implement these models in clinical practice.
The current Women Informed to Screen Depending on Measures of Risk (WISDOM) clinical trial examining risk-stratified mammographic screening, and the work by the group in Manchester, United Kingdom, incorporating risk SNPs and mammographic density into the Tyrer–Cuzick multivariable model, among others, will provide data with which to assess the impact of these approaches.
- Social inequalities in risk and burden
Socioeconomic differences
In epidemiological studies, a positive association between socioeconomic status and breast cancer risk is well established. This is due in large part to different distributions by socioeconomic status of breast cancer risk factors such as parity, age at first birth, and use of hormone therapy. Other possible contributors include differences in screening practices across socioeconomic status [34].
Given the increasing proportion of breast cancer cases in low- and middle-income countries, as well as the changing patterns of risk factors in these countries, it is critical to identify feasible strategies to improve prevention and early detection in these settings.
Racial and ethnic variations
Racial differences in breast cancer incidence and mortality exist, and it has become increasingly clear that differences in the distribution of both individual risk factors and societal and contextual factors, as well as tumour biology, all contribute to this variation.
For example, from the United States National Cancer Institute’s Surveillance, Epidemiology, and End Results (SEER) programme, the 2007–2011 age-adjusted incidence rate (per 100 000) for breast cancer was 128 for non-Hispanic White women and 123 for African American women, but the age-adjusted mortality rate (per 100 000) was 21.7 for non-Hispanic White women and 30.6 for African American women. African American women have a higher prevalence of triple-negative breast cancers [35], for which outcomes are poorer, and this is a likely contributor to the higher SEER mortality rates. However, even among the subset of women diagnosed with similar early-stage disease, mortality rates were higher for African American women, indicating that other factors, such as differences in patterns of care [35], contribute as well (see “The enduring disparity in breast cancer mortality between Black and White women in the USA” in Chapter 4.6).
Prevention trials require large study populations and long follow-up periods, which makes them both costly and challenging to conduct. Therefore, preliminary data for prevention trials often come from biomarker modulation studies, or from evaluation of the effects of interventions on contralateral breast cancer events in breast cancer treatment trials. Colditz and Bohlke recently reviewed the evidence that acting on already established information about modifiable risk factors could substantially reduce breast cancer incidence in high-income countries ( Table 5.9.1 ) [36].
Table 5.9.1
Current strategies to prevent breast cancer.
Weight loss
There have not been compelling new data for weight loss, but Breast Cancer Weight Loss (BWEL) is a current randomized trial addressing the ability of a weight-loss intervention to prevent breast cancer recurrence [37]. If BWEL is successful, weight loss would probably be further targeted in a trial for breast cancer risk reduction.
Metformin, which is used for treatment of metabolic syndrome and diabetes, has been linked with lower risk of breast cancer in observational studies. In a pre-surgical trial in Italy, metformin taken before surgery decreased levels of Ki-67, a marker of breast tissue proliferation, in women with insulin resistance [38], but in a meta-analysis on metformin and cancer risk, after adjustment for BMI, no significant reduction in breast cancer incidence was observed [38].
Familial or other high-risk groups
Other medical interventions generally target women who have substantial risk of breast cancer. The duration of the effects of selective ER modulators, such as tamoxifen and raloxifene, on breast cancer prevention was estimated in a meta-analysis, which demonstrated a measurable reduction in breast cancer incidence that was greatest in the first 5 years of follow-up but also extended into years 5–10 of follow–up [39].
In the follow-up of the International Breast Cancer Intervention Study (IBIS) trial (tamoxifen vs placebo), the hazard ratio for the occurrence of all breast cancers in the tamoxifen group versus the placebo group in the first 10 years of follow-up was 0.72 (95% CI, 0.59–0.88) and after 10 years of follow-up was 0.69 (95% CI, 0.53–0.91) ( Fig. 5.9.7 ) [40]. The effect was observed for both ER-positive breast cancer and ductal carcinoma in situ, but not for triple-negative breast cancer. Aromatase inhibitors, both anastrozole and exemestane, have been shown to reduce breast cancer risk by about half [41]. There is a lack of proven strategies for reducing the risk of HER2-positive and triple-negative breast cancers.
Fig. 5.9.7
Cumulative incidence of breast cancer over time in the International Breast Cancer Intervention Study I (IBIS-I) trial, according to treatment group (tamoxifen or placebo) and duration of follow-up. Solid lines indicate all breast cancers, and dashed (more...)
The management of women at high risk based on predisposing mutations in cancer susceptibility genes includes risk-reducing mastectomies and premenopausal oophorectomies, which may reduce risk of ER-positive breast cancer and of ovarian cancer. The timing and advisability may be considered in a framework put forward by Tung et al. (see Chapter 6.5) [42].
Recent data indicating that RANK ligand is an essential molecule in the development of breast cancer in BRCA1 mutation carriers have led to an international chemoprevention trial evaluating the RANK ligand inhibitor denosumab in BRCA1 mutation carriers, led by the Austrian Breast and Colorectal Cancer Study Group (ABCSG). The next phase of trials will focus on bringing progress in cancer immunology to prevention.
Clues to the biology of breast cancer risk.
Mammographic density.
Some rights reserved. This work is available under the Creative Commons Attribution-NonCommercial-NoDerivs 3.0 IGO licence (CC BY-NC-ND 3.0 IGO; https://creativecommons.org/licenses/by-nc-nd/3.0/igo/ ). Under the terms of this licence, you may copy and redistribute the work for non-commercial purposes, provided the work is appropriately cited, as indicated below. In any use of this work, there should be no suggestion that WHO endorses any specific organization, products or services. The use of the WHO logo is not permitted.
- Cite this Page Hankinson SE, Polyak K, Garber JE, et al. Breast cancer: Multiple, often complex, risk factors. In: Wild CP, Weiderpass E, Stewart BW, editors. World Cancer Report: Cancer research for cancer prevention. Lyon (FR): International Agency for Research on Cancer; 2020. 5.9.
- PDF version of this title (32M)
In this Page
Other titles in this collection.
- IARC World Cancer Reports
Related information
- PMC PubMed Central citations
- PubMed Links to PubMed
Recent Activity
- Breast cancer - World Cancer Report Breast cancer - World Cancer Report
Your browsing activity is empty.
Activity recording is turned off.
Turn recording back on
Connect with NLM
National Library of Medicine 8600 Rockville Pike Bethesda, MD 20894
Web Policies FOIA HHS Vulnerability Disclosure
Help Accessibility Careers
An official website of the United States government
Official websites use .gov A .gov website belongs to an official government organization in the United States.
Secure .gov websites use HTTPS A lock ( Lock Locked padlock icon ) or https:// means you've safely connected to the .gov website. Share sensitive information only on official, secure websites.
- Publications
- Account settings
- Advanced Search
- Journal List

Cancer Progress and Priorities: Breast Cancer
Serena c houghton, susan e hankinson.
- Author information
- Article notes
- Copyright and License information
Corresponding author: Serena Houghton, Arnold House 436, 715 North Pleasant Street, Amherst, MA 01002, [email protected]
Authors’ Contributions
Conception and design: S.C. Houghton, S.E. Hankinson
Writing, review, and/or revision of the manuscript: S.C. Houghton, S.E. Hankinson
Study supervision: S.C. Houghton, S.E. Hankinson
Keywords: Breast cancer, risk factors, prognosis, survival rate, heterogeneity
Introduction
Breast cancer is the most commonly diagnosed invasive cancer among women both globally and within the United States and the number one cause of cancer-related death among women globally [ 1 , 2 ]. Less than one percent of diagnosed breast cancers occur in men [ 2 ] and, therefore, male breast cancer is not included in this report. Breast cancer is an etiologically and clinically heterogeneous disease. Many risk factors, primarily hormone related, have been identified and these associations can vary by breast cancer subtype. Survival has increased over the past few decades, with the introduction of screening mammography and improved treatments. However, progress has not been seen equally among all ethnicities/ races or with all breast cancer subtypes (e.g., triple-negative).
Descriptive Epidemiology
Breast cancer accounts for 25% of new cancer cases in women globally, with an estimated 2,088,849 female breast cancer cancers occurring worldwide in 2018 (46.3 per 100,000 women) [ 1 , 3 ]. Based on available data, incidence rates are highest in Australia, New Zealand, much of Europe, and North America, intermediate in South America and Eastern Europe, and lowest in the majority of Asia and Africa ( Figure 1 ) [ 3 ]. Within the United States, breast cancer accounts for 30% of female cancer diagnoses with an estimated 268,600 new invasive breast cancers and an additional 62,930 cases of in situ breast cancer documented in 2019 (124.7 per 100,000 women) [ 2 ]. One in eight women will be diagnosed with breast cancer during their lifetime [ 2 ]. Diagnosis is rare before the age of 40 (probability <1%), after which incidence rates increase until about age 70 (median age at diagnosis: 62 years), before decreasing ( Figure 2 ) [ 2 , 4 ].
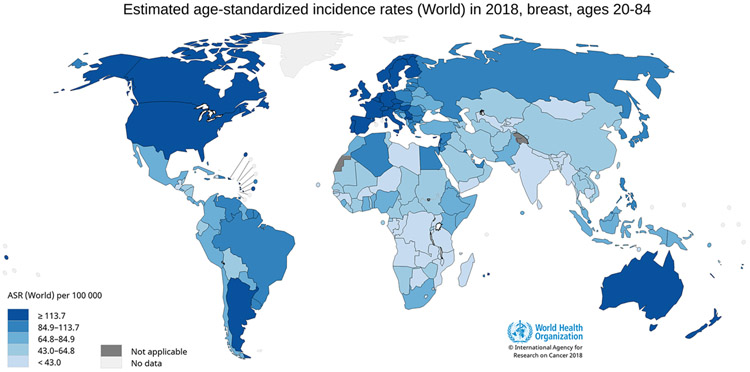
World age-standardized female breast cancer incidence rates for ages 20-84. Figure 1 shows age-standardized incidence rates for female breast cancer worldwide using data from GLOBOCAN, 2018. Breast cancer incidence is highest in Australia, New Zealand, Northern Europe, and North America, intermediate in Central and South America and Eastern Europe, and lowest in the majority of Asia and Africa. Data source: GLOBOCAN, 2018; Graph production: IARC ( http://gco.iarc.fr/today ) World Health Organization.
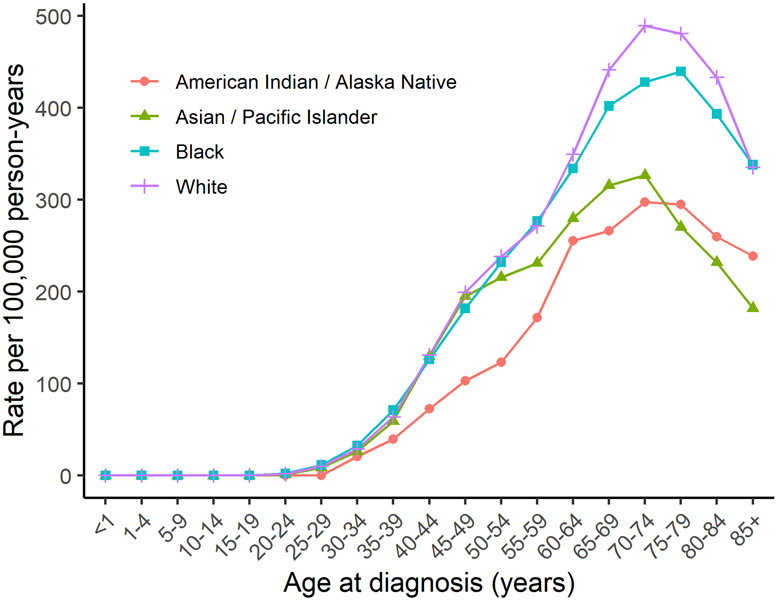
United States female breast cancer incidence rates by age of diagnosis and race/ethnicity. Figure 2 shows breast cancer incidence rates by age of diagnosis in the United States among White, Black, American Indian/ Alaskan Native, and Asian/ Pacific Islander women using data from the SEER program (SEER 21, 2013-2017).
In many westernized countries including the United States ( Figure 3 ), breast cancer incidence rates increased during the 1980s and 1990s due to changes in reproductive patterns, hormone therapy use, and increased mammographic screening [ 1 , 5 ]. Incidence rates then dropped in the early 2000s, particularly among women over 45 and for estrogen receptor-positive (ER) breast tumors, following a decline in hormone therapy use after the publication of the Women’s Health Initiative findings and declines in mammography screening rates [ 1 , 5 - 9 ]. Since 2004, incidence rates in the US have been increasing slowly (0.3% per year), potentially due to increasing obesity and declining birth rates [ 4 , 5 ]. Increases in ER+ tumors, particularly in situ, and decreases in ER− tumors are projected to continue according to forecasting models [ 9 - 11 ]. However, incidence rates have continued to decline or stabilize in multiple other westernized countries (e.g., Canada, UK, France, Australia) [ 1 , 8 ]. In contrast, incidence rates have been rapidly increasing in historically lower risk areas (e.g., Latin America, Africa, Asia) likely from increased life expectancy due to reductions in infectious diseases, increasing prevalence of overweight and obesity, changes in reproductive patterns, and increased breast cancer screening [ 1 , 8 ].
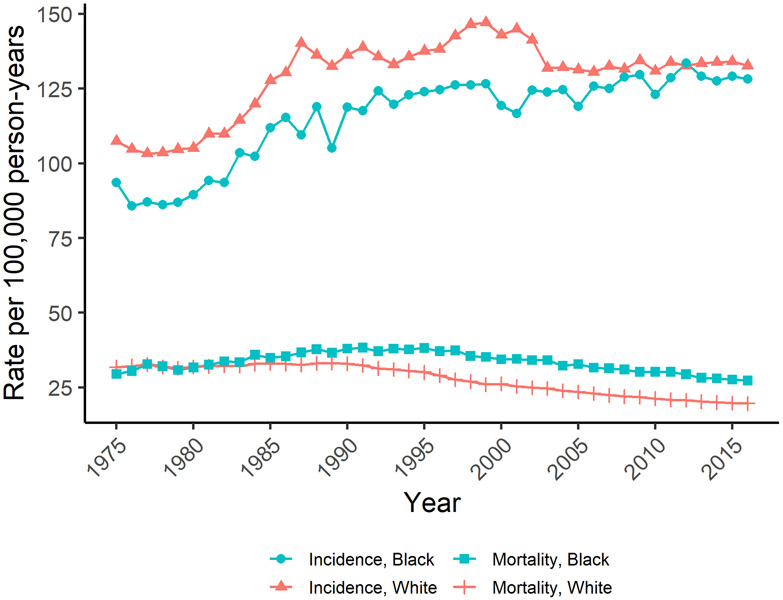
United States trends in age-adjusted female breast cancer incidence and mortality. Figure 3 shows trends in age-adjusted breast cancer incidence and mortality among White and Black United States women using data from the SEER program (SEER 9, 1975-2016).
Breast cancer is the most common cancer death in women globally, accounting for 15% of cancer deaths, with an estimated 626,700 breast cancer deaths among women in 2018 (13.0 per 100,000 women) [ 1 , 3 ]. Mortality rates are lowest in Eastern Asia (8.6 per 100,000 women) and highest in Fiji (36.9 per 100,000 women) ( Figure 4 ) [ 3 , 12 ]. In the US, where the lifetime risk of dying from breast cancer is 1 in 39 women, an estimated 41,760 women will die from breast cancer in 2019 (12.7 per 100,000 women) [ 5 , 12 ]. Mortality rates in the US increased between 1975 and 1989, then decreased through 2017 due to improvements in detection and treatment ( Figure 3 ) [ 5 , 13 ]. Similar trends have been observed in Canada and European countries [ 1 , 8 ], whereas mortality rates have increased in Asia, Africa, and Latin America [ 1 , 8 ].
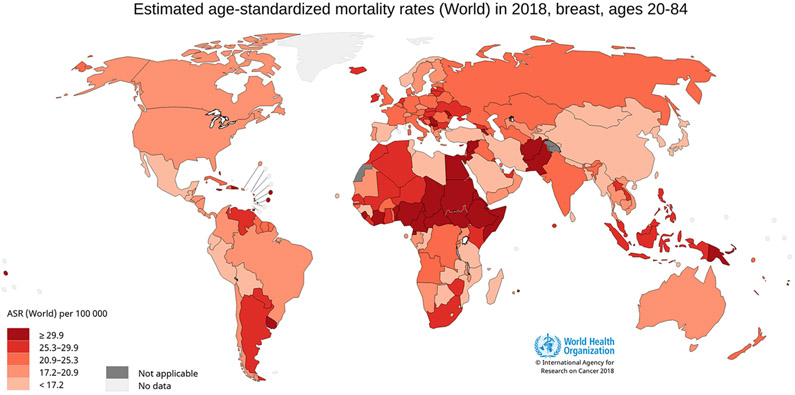
World age-standardized female breast cancer mortality rates for ages 20-84. Figure 4 shows age-standardized mortality rates for female breast cancer worldwide using data from GLOBOCAN, 2018. Breast cancer mortality is highest in parts of Africa and South-Eastern Asia, intermediate in Europe, and lowest in Eastern Asia. Data source: GLOBOCAN, 2018; Graph production: IARC ( http://gco.iarc.fr/today ) World Health Organization.
Survival among women with breast cancer
In the US, the 5-year relative survival is 91%, and after 10 and 15 years, the survival rates are 84% and 80%, respectively, for all stages combined [ 5 ]. The 5-year survival rate is 99% when the tumor is diagnosed at a local stage, 86% at a regional stage, and 17% when metastatic [ 5 ]. Survival rates by stage and subtype are shown in Figure 5 . Survival rates in the US have been increasing over time (74.8% in 1975 vs. 91.3% in 2015) likely due to earlier detection through mammographic screening and improved treatments such as the use of more targeted therapies [ 4 , 5 , 13 - 16 ].
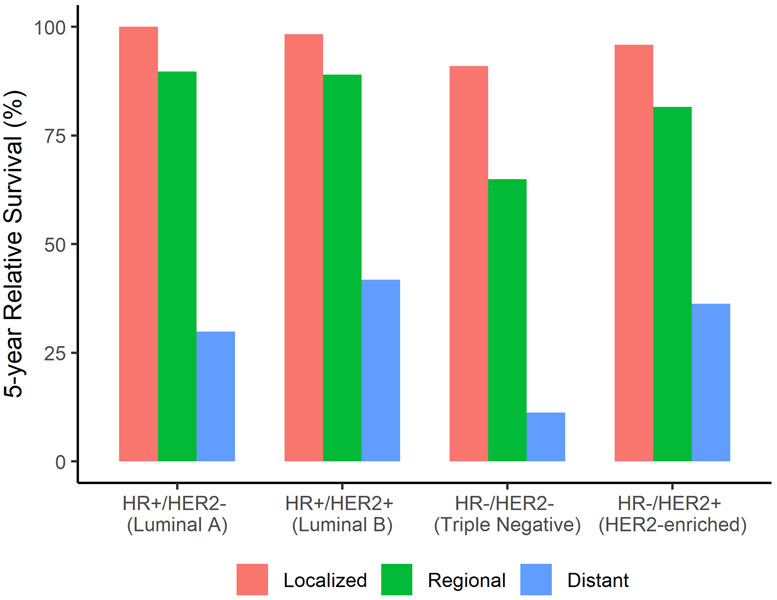
United States five-year relative female breast cancer subtype survival rates by stage at diagnosis. Figure 5 shows female breast cancer five-year relative survival rates for women at local, regional, and distant stages for each breast cancer subtype using data from the SEER program (SEER 18, 2000-2015).
Etiologic Heterogeneity
Breast cancer is a heterogeneous disease with considerable genetic and clinical heterogeneity [ 17 ]. Breast cancers, the majority of which are adenocarcinomas, are often classified by invasiveness (i.e., in situ or invasive), morphology, expression of immunohistochemical markers, and more recently through genetic panels. In turn, these features have been associated with differing responsiveness to treatment and prognosis [ 18 ]. In situ breast cancers are confined to the ducts or lobules [ 17 , 19 ]. Ductal carcinoma in situ (DCIS) is more common than lobular carcinoma in situ (LCIS), and while both are considered risk factors for invasive breast cancer, LCIS is not considered to be a lesion capable of becoming malignant [ 19 , 20 ]. However, the etiology and natural history of in situ tumors is not well known.
Invasive breast cancer is classified by histology, to guide clinical treatment, into invasive ductal (70-80% of breast cancer), invasive lobular (5-15% of breast cancer), and other less common types such as papillary tumors [ 17 , 19 ]. Immunohistochemical (IHC) staining for the estrogen receptor (ER), progesterone receptor (PR), and human epidermal growth factor receptor 2 (HER2) guide the use of targeted therapies. The use of other tests such as gene array profiling or other IHC markers are not as commonly used clinically [ 17 , 21 - 23 ]. Several main intrinsic molecular subtypes have been identified using gene micro-arrays: Luminal A, Luminal B, HER2-enriched, Basal Like, and Normal Like [ 17 , 24 - 26 ]. Further classifications of primarily triple negative tumors have identified the Claudin-low subtype and six triple-negative molecular subtypes [ 27 , 28 ]. Several gene panels (e.g., PAM50) have been developed as less expensive options with a similar ability to classify tumors into molecular subtypes [ 17 , 29 , 30 ]. In 2013, the St. Gallen consensus agreed on surrogate definitions of the intrinsic subtypes that could be approximated by IHC staining of ER, PR, and HER2 as well as grade and proliferation [ 31 ] ( Table 1 ), though several studies have noted that the agreement may be low [ 18 , 32 ].
Breast cancer intrinsic molecular subtypes
Sources: [ 17 - 19 , 31 ]
Disparities in the United States
Prior to age 40, US Black women have the highest breast cancer incidence rates, after which rates are highest among White women ( Figure 2 ). American Indian/ Alaska Native women have the lowest rates until age 74. Asian/ Pacific Islander women have similar incidence rates as White and Black women until age 45, after which they have the lowest incidence rates. Black women have the highest mortality rates at all ages followed by White women (e.g., 68.2 per 100,000 in Blacks vs 46.5 per 100,000 in Whites at age 60-64). Mortality rates for American Indian/ Alaska Native and Asian/ Pacific Islander women are similar until about age 60, at which point Asian/ Pacific Islander women have the lowest mortality rates (e.g., 30.0 per 100,000 in Asian/ Pacific Islander vs 33.2 per 100,000 in American Indian/ Alaska Native at age 60-64).
While breast cancer incidence rates have either declined or remained stable since the early 2000s among White women, incidence rates among Black women have continued to increase ( Figure 3 ) [ 33 ]. In 2016, the age-standardized incidence rate was 128.2 per 100,000 among Black women versus 132.7 per 100,000 among White women [ 4 ]. While incidence rates are lower, Black women experience higher breast cancer mortality than White women; further, that gap has continued to widen even as survival has increased overall ( Figure 3 ) [ 33 ]. The 2016 age-standardized mortality rate was 27.3 per 100,000 Black women versus 19.6 per 100,000 White women [ 4 ]. Compared to White women, Black women also have a higher incidence of the more aggressive triple-negative (ER−/PR−/HER2−) tumor subtype ( Figure 6 ) and, among those with ER+ tumors, Black women are more likely to be diagnosed at a later stage, potentially due to lower screening rates and barriers to health care access [ 33 - 35 ]. Further, disparities are still present within a similar stage and subtype of breast cancer [ 33 ]. For example, among women with ER+/HER2− tumors, Black women have lower survival at local (98.7% vs. 100.0%) and regional (82.2% vs. 90.6%) stages than White women, suggesting that racial disparities in treatment may play a role [ 4 , 33 ]. Studies have shown that Black women are more likely to experience delayed or inadequate surgery, radiation therapy, chemotherapy, and targeted therapies [ 33 , 35 - 37 ].
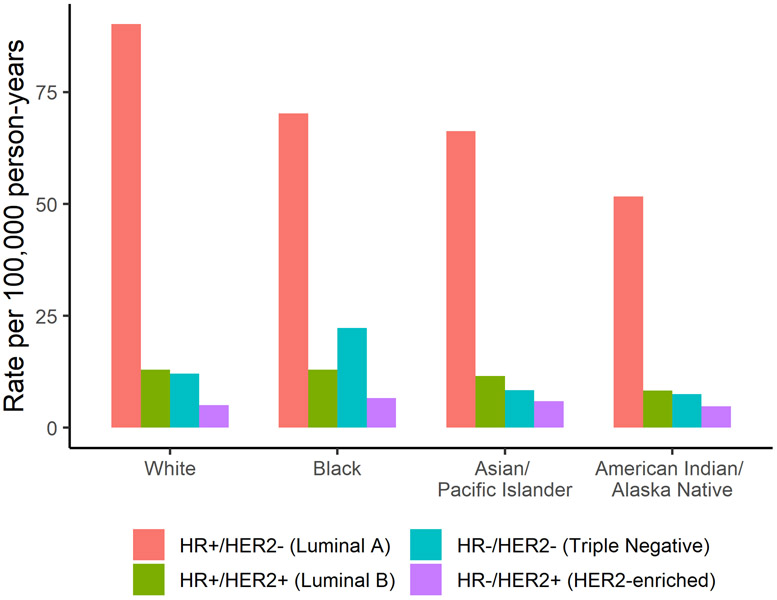
United States five-year age-adjusted female breast cancer incidence rates for each breast cancer subtype by race/ethnicity. Figure 6 shows five-year age-adjusted female breast cancer incidence rates by breast cancer subtype for White, Black, American Indian/ Alaskan Native, and Asian/ Pacific Islander women using data from the SEER program (SEER 21, 2012-2016).
Familial and genetic factors
Having a first-degree relative with breast cancer increases a woman’s risk of developing breast cancer two to three-fold [ 38 , 39 ]. Approximately 10-15% of breast cancers are thought to be hereditary, though a known pathogenic mutation is identified in only about 30% of those with hereditary breast cancer [ 40 ]. It is estimated that 5-10% of breast cancer is associated with a highly penetrant germ-line mutation, including in the BRCA1 and BRCA2 genes [ 41 ]. Those of Ashkenazi heritage (vs. without this heritage) have a higher prevalence of BRCA1/2 founder mutations (2.0-2.5% vs. 0.1-0.2%) [ 42 - 44 ]. A higher prevalence of BRCA1 mutations are found in women with early onset or triple-negative/ basal-like tumors ([ 45 ]). Additional genes with moderately and highly penetrant mutations associated with breast cancer are shown in Table 2 . Other similar genes that have been less consistently associated with breast cancer include BRIP1, BARD1, NBN, NF1, and RAD50 [ 46 - 51 ]. Several hundred common gene polymorphisms have been identified through genome-wide association studies (GWAS), which, cumulatively, currently explain about 18% of the two-fold familial relative risk [ 52 ].
Moderate to high penetrance genes associated with breast cancer
lobular breast cancer
Sources: [ 46 - 48 , 350 - 354 ]
Risk Factors
Breast cancer etiology is influenced by multiple exposures occurring over the life course, including early life factors during childhood and adolescence that can affect risk later in adulthood. Many of the risk factors identified, and some that still need to be elucidated by additional well-conducted research are shown in Table 3 . The approximate magnitude of risk associated with each of the established risk factors is presented in Figure 7 . Further, research has suggested that associations between breast cancer risk factors and breast cancer may vary by breast cancer subtype ( Table 4 ).
Summary of risk factors and serum biomarkers with breast cancer risk
ABBREVIATIONS: Refs, references; PL, pooled analysis; MA, meta-analysis; RCT, randomized trials; ER, estrogen receptor
NOTE: Color used to indicate consistency of association: Dark red, established/ probable positive association; light red, possible positive association; dark green, established/probable inverse association; light green, possible inverse association; grey, inconsistent/ no association.
Symbols used to indicate magnitude of association: + + + strong positive association (RR/OR >5); + + moderate positive association (RR/OR 2-5), + modest positive association (RR/OR <2); − − − strong inverse association (RR/OR <0.2); − − moderate inverse association (RR/OR 0.2-0.5), − modest inverse association (RR/OR >0.5).
Magnitude of association may vary by race/ethnicity, tumor subtype.
Association directions are for increasing exposure unless otherwise specified.
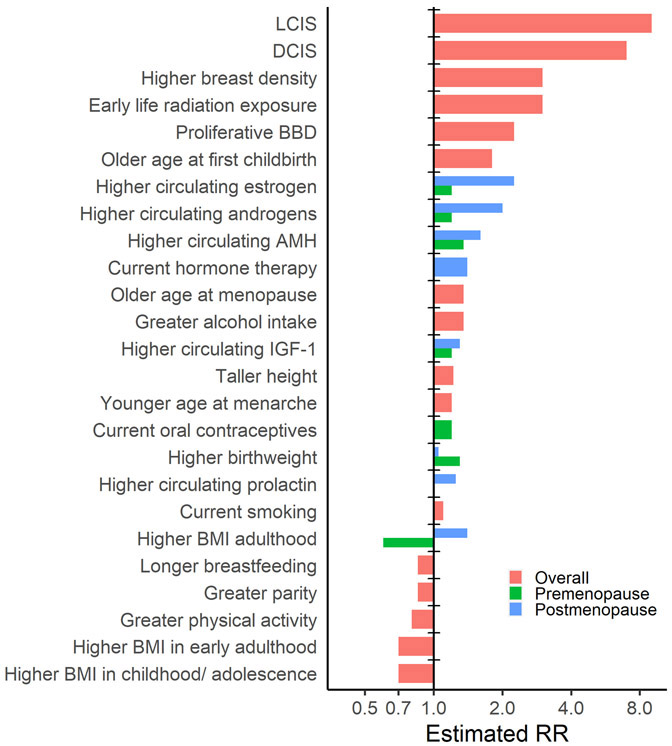
Magnitude of association for established breast cancer risk factors. Figure 7 shows the magnitude of association for each established breast cancer risk factor; if the association has been shown to consistently vary by menopause status both the premenopausal and postmenopausal associations are shown. Risks are approximate and can vary depending on the extent of exposure, breast cancer subtype, and menopause status.
Risk factors of breast cancer by subtypes
Sources [ 67 , 86 , 358 , 390 ]
Anthropometrics
The relationship between adiposity and breast cancer risk is complex and varies by the timing of body size assessment over the life course. Greater birthweight is associated with modestly higher risk of breast cancer in adulthood [ 53 , 54 ]. In contrast, a higher body mass index (BMI), indicating greater adiposity, measured in childhood or in early adulthood (18-30 years) is associated with decreased risk of breast cancer [ 55 - 63 ]. Premenopausal adult BMI is inversely associated with risk [ 61 , 63 - 66 ], while postmenopausal BMI is positively associated [ 63 - 66 ], particularly among never hormone therapy users and ER+ tumors [ 64 , 67 - 69 ]. The differing associations by menopausal status have been hypothesized to be due to the differences in estrogen levels and its primary sources (i.e., ovary vs. adipose tissue). In a large Mendelian randomization study using data from two breast cancer consortia, a polygenic risk score (PRS) of adult BMI was inversely associated with breast cancer risk regardless of menopausal status, suggesting the PRS may reflect early life obesity [ 70 ]. Larger central adiposity (e.g., waist circumference, waist-to-hip ratio) is associated with higher postmenopausal breast cancer risk and possibly greater risk of premenopausal breast cancer, though premenopausal studies have been limited and may be influenced by additional BMI-adjustment [ 63 , 71 ]. Adult weight gain is positively associated with postmenopausal breast cancer risk [ 63 , 72 , 73 ].
Other non-modifiable anthropometrics that increase breast cancer risk, particularly ER+ tumors, includes taller height [ 66 , 74 , 75 ] and larger birth lengths [ 53 , 76 ]. Having dense breasts, as assessed radiologically (e.g., digital mammogram), substantially increases risk regardless of menopausal status or hormone receptor status of the tumor [ 77 - 79 ]. For this reason, 38 states require breast density notifications after a mammogram, though language varies by state and may only state that there is an association in general and not contextualize the individual woman’s risk [ 80 ]. In March 2019, the FDA proposed a rule to extend this to all mammograms [ 80 ]. Associations have been much less consistent for bone mineral density. Early case-control studies observed increased risk; however, more recent meta-analyses of prospective studies do not see a significant association [ 81 , 82 ].
Reproductive factors
The associations of multiple reproductive factors with breast cancer risk have been well established. Younger age at menarche [ 83 ] and older age at menopause [ 83 ] are associated with increased breast cancer risk, potentially reflecting the number of ovulatory cycles over a woman's lifetime and estrogen exposure. Parous women initially have a higher risk of breast cancer after delivery compared to nulliparous women which peaks about 5 years after birth and remains elevated for approximately 20 years. Overall, women with greater parity have a lower risk of breast cancer long-term, and risk is further reduced with each subsequent birth [ 84 , 85 ]. However, this relationship does appear to vary by ER status [ 68 , 86 , 87 ]. Additionally, the younger age at which a woman has her first child [ 88 ] and longer durations of breastfeeding [ 84 ] further reduces breast cancer risk independent of parity.
Endogenous hormones and other circulating biomarkers
Sex hormones are integral in the etiology of breast cancer, supported by laboratory studies, epidemiologic evidence (e.g., reproductive risk factors and postmenopausal BMI) and the use of selective estrogen receptor modulators (e.g., Tamoxifen) to prevent breast cancer [ 89 , 90 ]. Higher circulating levels of estrogens [ 91 - 93 ], androgens [ 91 - 93 ], and prolactin [ 94 ], primarily in postmenopausal women, are established to increase breast cancer risk. Estrogen metabolites may also play a role [ 95 - 97 ] though evidence remains limited. Higher anti-mullerian hormone (AMH), measured premenopausally, is positively associated with breast cancer [ 98 ], though it is also strongly and directly related to age at menopause. Higher sex hormone binding globulin (SHBG) may decrease breast cancer risk [ 91 , 92 , 99 ]. Progesterone levels are not related to premenopausal breast cancer [ 91 ], possibly due to challenges in characterizing long-term hormone levels, and only one prospective study has examined postmenopausal progesterone levels, finding no association [ 100 ]. Circulating concentrations of insulin-like growth factor-1 are modestly positively associated with risk of ER+ breast cancer [ 101 ], which is further supported by a mendelian randomization study [ 102 ].
Other circulating biomarkers also may play a role in breast cancer etiology. Studies examining insulin or c-peptide, a byproduct of insulin, have suggested an increased risk among postmenopausal women only [ 103 - 107 ]. Potentially, chronic low levels of inflammation indicated by c-reactive protein may increase risk [ 108 - 110 ]. There is a suggestive increase in breast cancer risk for greater leptin and lower adiponectin levels [ 111 , 112 ]. Studies examining melatonin levels, which may be affected by light at night or shiftwork, have been relatively inconsistent perhaps due to differences in sample collection and timing, showing either inverse or null associations between the main melatonin metabolite, 6-sulfatoxymelatonin (aMT6s) in urine and breast cancer [ 113 , 114 ].
Exogenous hormones
Use of oral contraceptives increases breast cancer risk for up to 10 years after stopping use, and this is most consistently observed among current and recent users [ 115 - 117 ]. However, as oral contraceptive use occurs during the reproductive years, at ages when breast cancer incidence is low, the impact on population rates of breast cancer is minimal. Levonorgestrel-releasing intrauterine devices, also are suggestively associated with increased risk [ 118 ]. Other forms of hormonal contraceptives have been less studied.
The use of postmenopausal hormone therapy has been evaluated in multiple observational studies and randomized trials [ 119 ]. Combined estrogen and progestin use substantially increases risk, and associations are strongest for current/recent use and among those with the longest durations of use [ 119 ]. Long duration use of estrogen only is associated with more modest increases in risk; the major clinical trial did not observe increases in risk with estrogen only use, though timing of treatment (i.e. years after menopause) likely played a role [ 119 , 120 ]. Relatively few studies have examined doses, formulations, and changing use patterns.
There has been an interest in diet as a risk factor for breast cancer since early ecologic studies of fat and breast cancer mortality. Dietary fat has had considerable interest and controversy; however, most studies indicate no association overall with total fat [ 121 - 126 ]. Studies of carbohydrate intake have been inconsistent [ 127 ], though glycemic index/load may be associated with increased risk [ 127 , 128 ], and soluble fiber with decreased risk [ 129 - 131 ].
Assessments of specific foods have suggested an increased risk with processed meats [ 132 - 136 ] and decreases in risk with low-fat dairy [ 136 - 138 ] and fruit and vegetable intake [ 139 - 141 ]. Coffee and tea associations have been inconsistent, but there may be associations in subgroups [ 142 - 146 ]. Several nutrients have also been assessed as potential risk factors. Nutrients with suggestive decreased risk include calcium [ 147 - 149 ], vitamin D [ 150 - 153 ], and carotenoids, particularly β-carotene [ 154 - 157 ]. Higher heme-iron intake and plasma iron levels may increase risk [ 158 ]. However, most other nutrients are inconsistent or have not been found to be associated with breast cancer, including vitamin A [ 159 ], B-vitamins [ 160 , 161 ], vitamin C [ 159 , 162 , 163 ], vitamin E [ 159 ], folate [ 164 - 168 ], selenium [ 169 , 170 ], phytoestrogens [ 171 , 172 ], and isoflavones [ 173 - 176 ]. Additionally, multivitamins have not been associated with breast cancer risk [ 177 ]. Alcohol is the dietary factor most consistently associated with breast cancer, conferring a moderate increase in risk [ 178 - 183 ].
As foods and nutrients are not eaten in isolation and interactions between nutrients and foods are likely (e.g., folate and alcohol), the examination of dietary patterns is important. Some studies have suggested that dietary patterns such as the “prudent”, “western”, and Mediterranean may be associated with breast cancer [ 184 - 188 ]. Apart from the Mediterranean pattern, including olive oil, decreasing risk [ 189 ], studies are inconsistent or weakly associated, and there may be substantial confounding with other lifestyle risk factors.
Environmental
Although many environmental factors have been evaluated, most have limited or inconsistent evidence linking them to breast cancer, leading to some controversy. Obtaining valid exposure measures during susceptible periods of life continues to be a challenge. Exposure to secondhand smoke has been suggestively associated with increases in breast cancer risk [ 190 - 193 ]. Others, such as air pollution [ 194 , 195 ], electromagnetic fields [ 196 - 198 ], organochlorines (e.g., DDT/DDE, PCBs) [ 199 - 202 ], and acrylamides in food [ 203 ] have been inconsistent. Non-medical radiation exposure (e.g., atomic bombs) has been associated with increased breast cancer risk, particularly among those exposed at younger ages [ 204 , 205 ].
Lifestyle factors
Higher levels of physical activity have been consistently linked with decreases in breast cancer risk [ 63 , 206 - 210 ] and greater sedentary behavior or physical inactivity may be associated with increased risk [ 63 , 211 , 212 ]. Smoking has recently become quite well established to increase breast cancer risk, particularly if initiated prior to first pregnancy and for long durations [ 182 , 190 , 213 , 214 ]. The use of hair dye or relaxers may possibly be associated with increased risk among Black women in particular [ 215 , 216 ]. Exposure to light at night or shift work has been suggested to be positively associated with breast cancer, but the mechanism is unclear [ 217 - 221 ]. Sleeping duration is unlikely to be associated with risk [ 220 , 222 , 223 ]. Stressful life events may also be associated with increased risk, though definitions of stress or stressful life events have varied widely [ 224 ].
Medications/ other health conditions
The association of many medications with breast cancer risk (e.g., antibiotics [ 225 ], antidepressants [ 226 ], aspirin/NSAIDs [ 227 - 229 ], bisphosphonates [ 230 - 232 ], infertility drugs [ 233 , 234 ], statins [ 235 ]) has been inconsistent or null, and likely limited by confounding or ascertainment biases. Diethylstilbestrol (DES) has been found to increase breast cancer risk among women who took the drug during pregnancy [ 236 - 240 ], and associations among the daughters exposed in utero are less consistent but also may be positive [ 76 , 239 , 241 - 244 ]. Early case-control studies on abortion reported an increased risk; however, prospective studies have overall observed no association [ 245 - 248 ]. Prior history of breast conditions such as proliferative benign breast disease [ 249 , 250 ] or in situ tumors [ 251 - 260 ] has been established to substantially increase breast cancer. Obesity-related disorders, such as diabetes [ 261 - 265 ] and metabolic syndrome [ 266 ], have been generally inconsistent. Migraine headaches are likely not related, as observed associations have been limited to case-control studies [ 267 , 268 ]. Lastly, ionizing radiation for medical reasons (e.g., for lymphoma) has been established to increase breast cancer risk, which increases with greater doses and the highest risk occurs among those exposed before puberty similar to those exposed due to radiation from atomic bombs [ 204 , 205 , 269 ].
Other factors
Additional risk factors not included in Table 3 with very limited or insufficient evidence include a number of chemical or biologic agents (i.e., polycyclic aromatic hydrocarbons [ 270 ], parabens [ 271 ], BPA [ 272 , 273 ], phthalates [ 274 - 276 ], perfluorocarbons[ 277 - 279 ], human papillomavirus [ 280 ], Epstein–Barr virus [ 281 ]), breast size [ 282 ], blood pressure [ 283 - 287 ], under-wire bras [ 288 , 289 ], breast implants [ 290 ], cellphone use [ 291 ], deodorant/ antiperspirant use [ 292 - 294 ], and trauma to the breast [ 295 , 296 ].
Risk Prediction Models
Multiple risk prediction models have been developed to predict future risk of breast cancer (e.g., Gail/ Breast Cancer Risk Assessment Tool [BCRAT], Breast Cancer Surveillance Consortium [BCSC], Rosner-Colditz, Claus, BRCAPRO, Breast and Ovarian Analysis of Disease Incidence and Carrier Estimation Algorithm [BOADICEA], Tyrer-Cuszik/ International Breast Cancer Study [IBIS]), some of which can also predict BRCA carrier status (e.g., BRCAPRO, BOADICEA, IBIS) [ 297 , 298 ]. Risk prediction models are increasingly being used clinically to help guide decisions related to screening and prevention (e.g., timing and frequency of screening, use of chemoprevention) [ 299 - 301 ]. Risk prediction models vary in terms of factors included, with several including hormonal, environmental, or pathologic factors, high-risk genetic mutations, and more recently mammographic density [ 297 , 298 ]. While all models include family history assessment, the level of detail varies widely ranging from only first-degree relatives with breast cancer to all relatives with breast, ovarian, pancreas, and prostate cancers and their ages of onset [ 297 , 298 ]. The risk prediction models also vary in terms of type of population the model is suitable for (i.e., general screening population, women with a family history of breast or ovarian cancer) [ 297 , 298 ].
The Gail, Rosner-Colditz, Claus, BCSC, BRCAPRO, BOADICEA, and IBIS models have all been validated in external datasets [ 298 , 302 , 303 ]. A recent comparison of several of the most commonly used clinical models (i.e., Gail, BRCAPRO, BCSC, Claus, IBIS) in a large, predominantly White US screening population indicated that the models were generally well-calibrated (O/E range: 0.78-0.97) but with only moderate discrimination (AUC range: 0.61-0.64) [ 304 ]. Expansion of these models to include multiple biomarkers (e.g., mammographic density and/or other imaging features, polygenic risk scores, endogenous hormones, epigenetics, metabolomics), and the development and validation of models across race/ethnicity and by tumor subtype is ongoing, and likely to lead to model improvement [ 305 - 316 ].
Modifiable risk factors often targeted for breast cancer prevention include maintaining a healthy weight, participating in regular physical activity, moderating or avoiding alcohol intake, and minimizing or avoiding postmenopausal hormone therapy [ 317 ]. Women who most adhered to the American Cancer Society prevention guidelines had a 22% lower risk of breast cancer compared to women with the lowest adherence [ 318 ]. Other risk factors that could be targeted for prevention include healthy eating (e.g., increased intake of fruits and vegetables) [ 319 ] and, when possible, breastfeeding [ 320 ]. For example, among African American women, who often have lower rates of breastfeeding and higher rates of triple-negative breast cancer [ 317 , 321 ], facilitating increased breastfeeding may importantly lower the risk of triple-negative breast cancers [ 86 , 322 - 324 ]. Additionally, prevention programs (e.g., avoidance of smoking) in adolescents and young adults, particularly prior to first pregnancy, may be important, as these have been shown to be etiologically important periods.
For high-risk women, the American Society of Clinical Oncology recommends the use of selective estrogen receptor modulators (e.g., Tamoxifen, Raloxifene) or aromatase inhibitors (Exemestane, Anastrozole) [ 300 ]. While no single threshold for being high risk has been defined, women who are most likely to benefit from endocrine therapy are those with one or more of the following: diagnosis of atypical hyperplasia or LCIS, an estimated 5-year risk ≥3% (BCRAT) or 10-year risk ≥5% (IBIS), or a relative risk ≥4 times the population risk for ages 40-44 years or ≥2 times the population risk for ages 45-69 years. Tamoxifen can be used regardless of menopausal status, whereas Raloxifene, Exemestane, and Anastrozole can only be used by postmenopausal women [ 300 ]. Trials of Tamoxifen and Raloxifene have shown a 50% reduction in breast cancer risk, primarily due to a reduction of hormone receptor-positive breast cancers [ 317 , 325 - 327 ] and trials of aromatase inhibitors have shown similar reductions [ 328 , 329 ]. However, less than 10% of women eligible for chemoprevention use these drugs [ 330 ], primarily due to lack of provider recommendations and concerns about potential side effects. In the future, better targeting of women who may benefit, the use of newer selective estrogen receptor modulators, or low-dose of topical Tamoxifen may help mitigate these issues [ 331 - 333 ].
Additionally, in recent years there has been an increase in women electing to undergo surgical prevention for breast cancer [ 334 ]. The NCCN recommends prophylactic salpingo-oophorectomy for women with a known BRCA1/2 mutation between the ages of 35-40 that have completed childbearing and that prophylactic mastectomy should be discussed as an option for risk reduction (NCCN guidelines high risk). Bilateral prophylactic mastectomy has been shown to reduce breast cancer risk and increase survival [ 335 ]. Contralateral prophylactic mastectomy after the diagnosis of cancer in the other breast, has also been shown to reduce the breast cancer risk in the other breast; however, evidence for increased survival remains limited [ 335 ]. Prophylactic bilateral oophorectomy, the removal of both ovaries (generally with bilateral salpingectomy) reduces the risk of ovarian cancer and may also reduce the risk of breast cancer, though this is less clear [ 336 ].
Screening and Early Detection
Mammography is the most common modality for breast cancer screening in the United States; however, organizations vary in the recommended screening age range and frequency of mammograms. For example, for average-risk women, the US Preventive Services Task Force and the American Academy of Family Physicians now recommend biennial screening for women 50-74 years. In contrast, the American College of Radiology and the National Comprehensive Cancer Network recommend annual screening starting at age 40 [ 337 - 339 ]. In other high-income countries, the screening age range is most commonly 50 to 69 or 70 with a two-year screening interval [ 337 , 340 ]. However, in 2018 only 58-74% of women ages 50-74 had at least one screening mammogram in the prior two years [ 341 ]. Mammographic screening can identify breast cancer at earlier, more treatable stages, and thus reduce breast cancer mortality [ 337 , 342 , 343 ]. However, evidence has been inconsistent on whether this has occurred in all age groups, and concerns exist that screening increases the number of false positives, and may lead to the over detection of breast cancer [ 342 , 344 ]. Due to the difficulty of defining and estimating over detection, estimates have ranged widely from 1 to 60% in trials and 1–12% in studies with a low risk of bias [ 342 ]. While several studies have suggested that breast cancer mortality rates and advanced breast cancer diagnoses have been reduced with screening mammography [ 343 ], others have suggested that other factors are additionally responsible (e.g., better treatments) [ 344 ]. Among women with a 20% or greater lifetime risk of breast cancer, several organizations recommend annual supplemental MRIs [ 337 ]. Additionally, as mammography is less sensitive for women with dense breasts [ 345 ], alternative and supplemental screenings have been proposed including more frequent mammograms, supplemental ultrasound, MRI, and digital breast tomosynthesis (i.e., 3D mammography) [ 337 , 345 ]. Several trials are currently underway examining breast cancer screening intervals and start ages (i.e., WISDOM) and the use of digital breast tomosynthesis or ultrasound (i.e., TMIST, DBTUST, ASTOUND) [ 346 - 349 ].
Future Directions
While earlier detection and improved treatments have reduced breast cancer mortality, breast cancer continues to be the most common cancer among women, and incidence is projected to continue to increase in the next few decades. To combat this, multiple strategies are needed. Further research into etiologic heterogeneity should be conducted, such as risk factors among different ethnicities and tumor subtypes, particularly for ER-negative/ basal-like tumors where fewer effective treatments are currently available. Underlying mechanisms of known risk factors should be examined, including reasons for heterogeneity by menopausal status or tumor subtype, potentially using emerging technologies (e.g., metabolomics, proteomics) to assess local and systemic biomarkers and tumor heterogeneity. Further improvement in and validation of risk prediction models is needed, for example, by the addition of both biomarkers (e.g., breast imaging, genetics, hormones) and lifestyle factors and further development of models that better model risk at both the youngest and oldest ages, among different ethnicities, and for subtypes particularly ER−, for which models perform less well. Finally, additional efforts to determine how to successfully implement known (e.g., weight maintenance or reduction) and future preventive strategies, during susceptible periods, is critical.
Continued research on alternative screening modalities that may increase adherence or be more effective (e.g., more sensitive and specific) is needed. In addition to increasing prevention activities and awareness, further development or trials of chemopreventives with lower doses or better side effect profiles to increase adherence or uptake when appropriate and chemopreventives for ER-negative breast cancers may be beneficial. In addition to improvements in screening and treatments, research on improving access and equity in cancer care is needed to address existing disparities. Lastly, with the observed increases in breast cancer survival rates over time, additional research is needed regarding survivorship to improve quality of life.
Acknowledgments
Financial support: SCH was supported through National Research Service Awards F32 CA224677 by the National Cancer Institute. SEH was supported through R01 CA207369 by the National Cancer Institute.
Disclosure of Potential Conflicts of Interest: No potential conflicts of interest were disclosed.
- 1. American Cancer Society (2018) Global Cancer Facts & Figures 4th Edition. American Cancer Society, Atlanta, GA [ Google Scholar ]
- 2. Siegel RL, Miller KD, Jemal A (2019) Cancer statistics, 2019. CA Cancer J Clin 69:7–34. 10.3322/caac.21551 [ DOI ] [ PubMed ] [ Google Scholar ]
- 3. Bray F, Ferlay J, Soerjomataram I, et al. (2018) Global cancer statistics 2018: GLOBOCAN estimates of incidence and mortality worldwide for 36 cancers in 185 countries. CA Cancer J Clin 68:394–424. 10.3322/caac.21492 [ DOI ] [ PubMed ] [ Google Scholar ]
- 4. SEER*Explorer. In: SEER. https://seer.cancer.gov/explorer/index.html . Accessed 16 Dec 2019 [ Google Scholar ]
- 5. American Cancer Society (2019) Breast Cancer Facts & Figures 2019-2020. American Cancer Society, Atlanta, GA [ Google Scholar ]
- 6. Kerlikowske K, Miglioretti DL, Buist DSM, et al. (2007) Declines in invasive breast cancer and use of postmenopausal hormone therapy in a screening mammography population. J Natl Cancer Inst 99:1335–1339. 10.1093/jnci/djm111 [ DOI ] [ PubMed ] [ Google Scholar ]
- 7. Chlebowski RT, Kuller LH, Prentice RL, et al. (2009) Breast cancer after use of estrogen plus progestin in postmenopausal women. N Engl J Med 360:573–587. 10.1056/NEJMoa0807684 [ DOI ] [ PMC free article ] [ PubMed ] [ Google Scholar ]
- 8. Torre LA, Islami F, Siegel RL, et al. (2017) Global Cancer in Women: Burden and Trends. Cancer Epidemiol Biomark Prev Publ Am Assoc Cancer Res Cosponsored Am Soc Prev Oncol 26:444–457. 10.1158/1055-9965.EPI-16-0858 [ DOI ] [ PubMed ] [ Google Scholar ]
- 9. Glass AG, Lacey JV, Carreon JD, Hoover RN (2007) Breast cancer incidence, 1980-2006: combined roles of menopausal hormone therapy, screening mammography, and estrogen receptor status. J Natl Cancer Inst 99:1152–1161. 10.1093/jnci/djm059 [ DOI ] [ PubMed ] [ Google Scholar ]
- 10. Anderson WF, Katki HA, Rosenberg PS (2011) Incidence of breast cancer in the United States: current and future trends. J Natl Cancer Inst 103:1397–1402. 10.1093/jnci/djr257 [ DOI ] [ PMC free article ] [ PubMed ] [ Google Scholar ]
- 11. Rosenberg PS, Barker KA, Anderson WF (2015) Estrogen Receptor Status and the Future Burden of Invasive and In Situ Breast Cancers in the United States. J Natl Cancer Inst 107:. 10.1093/jnci/djv159 [ DOI ] [ PMC free article ] [ PubMed ] [ Google Scholar ]
- 12. GLOBOCAN. http://gco.iarc.fr/today/home . Accessed 16 Dec 2019
- 13. Berry DA, Cronin KA, Plevritis SK, et al. (2005) Effect of screening and adjuvant therapy on mortality from breast cancer. N Engl J Med 353:1784–1792. 10.1056/NEJMoa050518 [ DOI ] [ PubMed ] [ Google Scholar ]
- 14. Kristeleit H, Parton M, Beresford M, et al. (2016) Long-term Follow-up Data from Pivotal Studies of Adjuvant Trastuzumab in Early Breast Cancer. Target Oncol 11:579–591. 10.1007/s11523-016-0438-5 [ DOI ] [ PMC free article ] [ PubMed ] [ Google Scholar ]
- 15. (2015) Aromatase inhibitors versus tamoxifen in early breast cancer: patient-level meta-analysis of the randomised trials. The Lancet 386:1341–1352. 10.1016/S0140-6736(15)61074-1 [ DOI ] [ PubMed ] [ Google Scholar ]
- 16. (2005) Effects of chemotherapy and hormonal therapy for early breast cancer on recurrence and 15-year survival: an overview of the randomised trials. The Lancet 365:1687–1717. 10.1016/S0140-6736(05)66544-0 [ DOI ] [ PubMed ] [ Google Scholar ]
- 17. Malhotra GK, Zhao X, Band H, Band V (2010) Histological, molecular and functional subtypes of breast cancers. Cancer Biol Ther 10:955–960. 10.4161/cbt.10.10.13879 [ DOI ] [ PMC free article ] [ PubMed ] [ Google Scholar ]
- 18. Prat A, Pineda E, Adamo B, et al. (2015) Clinical implications of the intrinsic molecular subtypes of breast cancer. Breast Edinb Scotl 24 Suppl 2:S26–35. 10.1016/j.breast.2015.07.008 [ DOI ] [ PubMed ] [ Google Scholar ]
- 19. Makki J (2015) Diversity of Breast Carcinoma: Histological Subtypes and Clinical Relevance. Clin Med Insights Pathol 8:23–31. 10.4137/CPath.S31563 [ DOI ] [ PMC free article ] [ PubMed ] [ Google Scholar ]
- 20. Giuliano AE, Connolly JL, Edge SB, et al. (2017) Breast Cancer—Major changes in the American Joint Committee on Cancer eighth edition cancer staging manual. CA Cancer J Clin 67:290–303. 10.3322/caac.21393 [ DOI ] [ PubMed ] [ Google Scholar ]
- 21. Gradishar WJ, Anderson BO, Balassanian R, et al. (2018) Breast Cancer, Version 4.2017, NCCN Clinical Practice Guidelines in Oncology. J Natl Compr Canc Netw 16:310–320. 10.6004/jnccn.2018.0012 [ DOI ] [ PubMed ] [ Google Scholar ]
- 22. Lester T, Wang J, Bourne P, et al. (2009) Different panels of markers should be used to predict mammary Paget’s disease associated with in situ or invasive ductal carcinoma of the breast. Ann Clin Lab Sci 39:17–24 [ PubMed ] [ Google Scholar ]
- 23. Walker LC, Harris GC, Holloway AJ, et al. (2007) Cytokeratin KRT8/18 expression differentiates distinct subtypes of grade 3 invasive ductal carcinoma of the breast. Cancer Genet Cytogenet 178:94–103. 10.1016/j.cancergencyto.2007.06.002 [ DOI ] [ PubMed ] [ Google Scholar ]
- 24. Perou CM, Sørlie T, Eisen MB, et al. (2000) Molecular portraits of human breast tumours. Nature 406:747–752. 10.1038/35021093 [ DOI ] [ PubMed ] [ Google Scholar ]
- 25. Sorlie T, Tibshirani R, Parker J, et al. (2003) Repeated observation of breast tumor subtypes in independent gene expression data sets. Proc Natl Acad Sci U S A 100:8418–8423. 10.1073/pnas.0932692100 [ DOI ] [ PMC free article ] [ PubMed ] [ Google Scholar ]
- 26. Sørlie T, Perou CM, Tibshirani R, et al. (2001) Gene expression patterns of breast carcinomas distinguish tumor subclasses with clinical implications. Proc Natl Acad Sci U S A 98:10869–10874. 10.1073/pnas.191367098 [ DOI ] [ PMC free article ] [ PubMed ] [ Google Scholar ]
- 27. Prat A, Parker JS, Karginova O, et al. (2010) Phenotypic and molecular characterization of the claudin-low intrinsic subtype of breast cancer. Breast Cancer Res BCR 12:R68. 10.1186/bcr2635 [ DOI ] [ PMC free article ] [ PubMed ] [ Google Scholar ]
- 28. Lehmann BD, Bauer JA, Chen X, et al. (2011) Identification of human triple-negative breast cancer subtypes and preclinical models for selection of targeted therapies. J Clin Invest 121:2750–2767. 10.1172/JCI45014 [ DOI ] [ PMC free article ] [ PubMed ] [ Google Scholar ]
- 29. Parker JS, Mullins M, Cheang MCU, et al. (2009) Supervised risk predictor of breast cancer based on intrinsic subtypes. J Clin Oncol Off J Am Soc Clin Oncol 27:1160–1167. 10.1200/JCO.2008.18.1370 [ DOI ] [ PMC free article ] [ PubMed ] [ Google Scholar ]
- 30. Ross JS, Hatzis C, Symmans WF, et al. (2008) Commercialized multigene predictors of clinical outcome for breast cancer. The Oncologist 13:477–493. 10.1634/theoncologist.2007-0248 [ DOI ] [ PubMed ] [ Google Scholar ]
- 31. Goldhirsch A, Winer EP, Coates AS, et al. (2013) Personalizing the treatment of women with early breast cancer: highlights of the St Gallen International Expert Consensus on the Primary Therapy of Early Breast Cancer 2013. Ann Oncol Off J Eur Soc Med Oncol 24:2206–2223. 10.1093/annonc/mdt303 [ DOI ] [ PMC free article ] [ PubMed ] [ Google Scholar ]
- 32. Lundgren C, Bendahl P-O, Borg Å, et al. (2019) Agreement between molecular subtyping and surrogate subtype classification: a contemporary population-based study of ER-positive/HER2-negative primary breast cancer. Breast Cancer Res Treat 178:459–467. 10.1007/s10549-019-05378-7 [ DOI ] [ PMC free article ] [ PubMed ] [ Google Scholar ]
- 33. Reeder-Hayes KE, Anderson BO (2017) Breast Cancer Disparities at Home and Abroad: A Review of the Challenges and Opportunities for System-Level Change. Clin Cancer Res Off J Am Assoc Cancer Res 23:2655–2664. 10.1158/1078-0432.CCR-16-2630 [ DOI ] [ PMC free article ] [ PubMed ] [ Google Scholar ]
- 34. Ahmed AT, Welch BT, Brinjikji W, et al. (2017) Racial Disparities in Screening Mammography in the United States: A Systematic Review and Meta-analysis. J Am Coll Radiol JACR 14:157–165.e9. 10.1016/j.jacr.2016.07.034 [ DOI ] [ PubMed ] [ Google Scholar ]
- 35. Yedjou CG, Tchounwou PB, Payton M, et al. (2017) Assessing the Racial and Ethnic Disparities in Breast Cancer Mortality in the United States. Int J Environ Res Public Health 14:. 10.3390/ijerph14050486 [ DOI ] [ PMC free article ] [ PubMed ] [ Google Scholar ]
- 36. Green AK, Aviki EM, Matsoukas K, et al. (2018) Racial disparities in chemotherapy administration for early-stage breast cancer: a systematic review and meta-analysis. Breast Cancer Res Treat 172:247–263. 10.1007/s10549-018-4909-5 [ DOI ] [ PMC free article ] [ PubMed ] [ Google Scholar ]
- 37. Roberts MC, Weinberger M, Dusetzina SB, et al. (2016) Racial Variation in the Uptake of Oncotype DX Testing for Early-Stage Breast Cancer. J Clin Oncol Off J Am Soc Clin Oncol 34:130–138. 10.1200/JCO.2015.63.2489 [ DOI ] [ PMC free article ] [ PubMed ] [ Google Scholar ]
- 38. Shiyanbola OO, Arao RF, Miglioretti DL, et al. (2017) Emerging Trends in Family History of Breast Cancer and Associated Risk. Cancer Epidemiol Biomark Prev Publ Am Assoc Cancer Res Cosponsored Am Soc Prev Oncol 26:1753–1760. 10.1158/1055-9965.EPI-17-0531 [ DOI ] [ PMC free article ] [ PubMed ] [ Google Scholar ]
- 39. Collaborative Group on Hormonal Factors in Breast Cancer (2001) Familial breast cancer: collaborative reanalysis of individual data from 52 epidemiological studies including 58,209 women with breast cancer and 101,986 women without the disease. Lancet Lond Engl 358:1389–1399. 10.1016/S0140-6736(01)06524-2 [ DOI ] [ PubMed ] [ Google Scholar ]
- 40. Samadder NJ, Giridhar KV, Baffy N, et al. (2019) Hereditary Cancer Syndromes-A Primer on Diagnosis and Management: Part 1: Breast-Ovarian Cancer Syndromes. Mayo Clin Proc 94:1084–1098. 10.1016/j.mayocp.2019.02.017 [ DOI ] [ PubMed ] [ Google Scholar ]
- 41. Bennett IC, Gattas M, Teh BT (1999) The genetic basis of breast cancer and its clinical implications. Aust N Z J Surg 69:95–105. 10.1046/j.1440-1622.1999.01515.x [ DOI ] [ PubMed ] [ Google Scholar ]
- 42. Ford D, Easton DF, Peto J (1995) Estimates of the gene frequency of BRCA1 and its contribution to breast and ovarian cancer incidence. Am J Hum Genet 57:1457–1462 [ PMC free article ] [ PubMed ] [ Google Scholar ]
- 43. Oddoux C, Struewing JP, Clayton CM, et al. (1996) The carrier frequency of the BRCA2 6174delT mutation among Ashkenazi Jewish individuals is approximately 1%. Nat Genet 14:188–190. 10.1038/ng1096-188 [ DOI ] [ PubMed ] [ Google Scholar ]
- 44. Struewing JP, Abeliovich D, Peretz T, et al. (1995) The carrier frequency of the BRCA1 185delAG mutation is approximately 1 percent in Ashkenazi Jewish individuals. Nat Genet 11:198–200. 10.1038/ng1095-198 [ DOI ] [ PubMed ] [ Google Scholar ]
- 45. Hahnen E, Hauke J, Engel C, et al. (2017) Germline Mutations in Triple-Negative Breast Cancer. Breast Care 12:15–19. 10.1159/000455999 [ DOI ] [ PMC free article ] [ PubMed ] [ Google Scholar ]
- 46. Easton DF, Pharoah PDP, Antoniou AC, et al. (2015) Gene-panel sequencing and the prediction of breast-cancer risk. N Engl J Med 372:2243–2257. 10.1056/NEJMsr1501341 [ DOI ] [ PMC free article ] [ PubMed ] [ Google Scholar ]
- 47. Hauke J, Horvath J, Groß E, et al. (2018) Gene panel testing of 5589 BRCA1/2-negative index patients with breast cancer in a routine diagnostic setting: results of the German Consortium for Hereditary Breast and Ovarian Cancer. Cancer Med 7:1349–1358. 10.1002/cam4.1376 [ DOI ] [ PMC free article ] [ PubMed ] [ Google Scholar ]
- 48. Couch FJ, Shimelis H, Hu C, et al. (2017) Associations Between Cancer Predisposition Testing Panel Genes and Breast Cancer. JAMA Oncol 3:1190–1196. 10.1001/jamaoncol.2017.0424 [ DOI ] [ PMC free article ] [ PubMed ] [ Google Scholar ]
- 49. Zhang B, Beeghly-Fadiel A, Long J, Zheng W (2011) Genetic variants associated with breast-cancer risk: comprehensive research synopsis, meta-analysis, and epidemiological evidence. Lancet Oncol 12:477–488. 10.1016/S1470-2045(11)70076-6 [ DOI ] [ PMC free article ] [ PubMed ] [ Google Scholar ]
- 50. Aloraifi F, Boland MR, Green AJ, Geraghty JG (2015) Gene analysis techniques and susceptibility gene discovery in non-BRCA1/BRCA2 familial breast cancer. Surg Oncol 24:100–109. 10.1016/j.suronc.2015.04.003 [ DOI ] [ PubMed ] [ Google Scholar ]
- 51. Angeli D, Salvi S, Tedaldi G (2020) Genetic Predisposition to Breast and Ovarian Cancers: How Many and Which Genes to Test? Int J Mol Sci 21:. 10.3390/ijms21031128 [ DOI ] [ PMC free article ] [ PubMed ] [ Google Scholar ]
- 52. Michailidou K, Lindström S, Dennis J, et al. (2017) Association analysis identifies 65 new breast cancer risk loci. Nature 551:92–94. 10.1038/nature24284 [ DOI ] [ PMC free article ] [ PubMed ] [ Google Scholar ]
- 53. Silva I dos S, De Stavola B, McCormack V, Collaborative Group on Pre-Natal Risk Factors and Subsequent Risk of Breast Cancer (2008) Birth size and breast cancer risk: re-analysis of individual participant data from 32 studies. PLoS Med 5:e193. 10.1371/journal.pmed.0050193 [ DOI ] [ PMC free article ] [ PubMed ] [ Google Scholar ]
- 54. Xu X, Dailey AB, Peoples-Sheps M, et al. (2009) Birth weight as a risk factor for breast cancer: a meta-analysis of 18 epidemiological studies. J Womens Health 2002 18:1169–1178. 10.1089/jwh.2008.1034 [ DOI ] [ PubMed ] [ Google Scholar ]
- 55. Warner ET, Hu R, Collins LC, et al. (2016) Height and Body Size in Childhood, Adolescence, and Young Adulthood and Breast Cancer Risk According to Molecular Subtype in the Nurses’ Health Studies. Cancer Prev Res Phila Pa 9:732–738. 10.1158/1940-6207.CAPR-16-0085 [ DOI ] [ PMC free article ] [ PubMed ] [ Google Scholar ]
- 56. Keinan-Boker L, Levine H, Derazne E, et al. (2016) Measured adolescent body mass index and adult breast cancer in a cohort of 951,480 women. Breast Cancer Res Treat 158:157–167. 10.1007/s10549-016-3860-6 [ DOI ] [ PubMed ] [ Google Scholar ]
- 57. Fagherazzi G, Guillas G, Boutron-Ruault M-C, et al. (2013) Body shape throughout life and the risk for breast cancer at adulthood in the French E3N cohort. Eur J Cancer Prev Off J Eur Cancer Prev Organ ECP 22:29–37. 10.1097/CEJ.0b013e328355ec04 [ DOI ] [ PubMed ] [ Google Scholar ]
- 58. Andersen ZJ, Baker JL, Bihrmann K, et al. (2014) Birth weight, childhood body mass index, and height in relation to mammographic density and breast cancer: a register-based cohort study. Breast Cancer Res BCR 16:R4. 10.1186/bcr3596 [ DOI ] [ PMC free article ] [ PubMed ] [ Google Scholar ]
- 59. Horn-Ross PL, Canchola AJ, Bernstein L, et al. (2016) Lifetime body size and estrogen-receptor-positive breast cancer risk in the California Teachers Study cohort. Breast Cancer Res BCR 18:132. 10.1186/s13058-016-0790-5 [ DOI ] [ PMC free article ] [ PubMed ] [ Google Scholar ]
- 60. Weiderpass E, Braaten T, Magnusson C, et al. (2004) A prospective study of body size in different periods of life and risk of premenopausal breast cancer. Cancer Epidemiol Biomark Prev Publ Am Assoc Cancer Res Cosponsored Am Soc Prev Oncol 13:1121–1127 [ PubMed ] [ Google Scholar ]
- 61. Premenopausal Breast Cancer Collaborative Group, Schoemaker MJ, Nichols HB, et al. (2018) Association of Body Mass Index and Age With Subsequent Breast Cancer Risk in Premenopausal Women. JAMA Oncol 4:e181771. 10.1001/jamaoncol.2018.1771 [ DOI ] [ PMC free article ] [ PubMed ] [ Google Scholar ]
- 62. Hidayat K, Yang C-M, Shi B-M (2018) Body fatness at a young age, body fatness gain and risk of breast cancer: systematic review and meta-analysis of cohort studies. Obes Rev Off J Int Assoc Study Obes 19:254–268. 10.1111/obr.12627 [ DOI ] [ PubMed ] [ Google Scholar ]
- 63. Chan DSM, Abar L, Cariolou M, et al. (2019) World Cancer Research Fund International: Continuous Update Project-systematic literature review and meta-analysis of observational cohort studies on physical activity, sedentary behavior, adiposity, and weight change and breast cancer risk. Cancer Causes Control CCC 30:1183–1200. 10.1007/s10552-019-01223-w [ DOI ] [ PubMed ] [ Google Scholar ]
- 64. Chen Y, Liu L, Zhou Q, et al. (2017) Body mass index had different effects on premenopausal and postmenopausal breast cancer risks: a dose-response meta-analysis with 3,318,796 subjects from 31 cohort studies. BMC Public Health 17:936. 10.1186/s12889-017-4953-9 [ DOI ] [ PMC free article ] [ PubMed ] [ Google Scholar ]
- 65. Liu K, Zhang W, Dai Z, et al. (2018) Association between body mass index and breast cancer risk: evidence based on a dose-response meta-analysis. Cancer Manag Res 10:143–151. 10.2147/CMAR.S144619 [ DOI ] [ PMC free article ] [ PubMed ] [ Google Scholar ]
- 66. van den Brandt PA, Spiegelman D, Yaun SS, et al. (2000) Pooled analysis of prospective cohort studies on height, weight, and breast cancer risk. Am J Epidemiol 152:514–527 [ DOI ] [ PubMed ] [ Google Scholar ]
- 67. Gaudet MM, Gierach GL, Carter BD, et al. (2018) Pooled Analysis of Nine Cohorts Reveals Breast Cancer Risk Factors by Tumor Molecular Subtype. Cancer Res 78:6011–6021. 10.1158/0008-5472.CAN-18-0502 [ DOI ] [ PMC free article ] [ PubMed ] [ Google Scholar ]
- 68. Yang XR, Chang-Claude J, Goode EL, et al. (2011) Associations of breast cancer risk factors with tumor subtypes: a pooled analysis from the Breast Cancer Association Consortium studies. J Natl Cancer Inst 103:250–263. 10.1093/jnci/djq526 [ DOI ] [ PMC free article ] [ PubMed ] [ Google Scholar ]
- 69. Suzuki R, Orsini N, Saji S, et al. (2009) Body weight and incidence of breast cancer defined by estrogen and progesterone receptor status—A meta-analysis. Int J Cancer 124:698–712. 10.1002/ijc.23943 [ DOI ] [ PubMed ] [ Google Scholar ]
- 70. Guo Y, Warren Andersen S, Shu X-O, et al. (2016) Genetically Predicted Body Mass Index and Breast Cancer Risk: Mendelian Randomization Analyses of Data from 145,000 Women of European Descent. PLoS Med 13:e1002105. 10.1371/journal.pmed.1002105 [ DOI ] [ PMC free article ] [ PubMed ] [ Google Scholar ]
- 71. Chen G-C, Chen S-J, Zhang R, et al. (2016) Central obesity and risks of pre- and postmenopausal breast cancer: a dose-response meta-analysis of prospective studies. Obes Rev Off J Int Assoc Study Obes 17:1167–1177. 10.1111/obr.12443 [ DOI ] [ PubMed ] [ Google Scholar ]
- 72. Teras LR, Patel AV, Wang M, et al. (2019) Sustained weight loss and risk of breast cancer in women ≥50 years: a pooled analysis of prospective data. J Natl Cancer Inst. 10.1093/jnci/djz226 [ DOI ] [ PMC free article ] [ PubMed ] [ Google Scholar ]
- 73. Schoemaker MJ, Nichols HB, Wright LB, et al. (2020) Adult weight change and premenopausal breast cancer risk: A prospective pooled analysis of data from 628,463 women. Int J Cancer. 10.1002/ijc.32892 [ DOI ] [ PMC free article ] [ PubMed ] [ Google Scholar ]
- 74. Wirén S, Häggström C, Ulmer H, et al. (2014) Pooled cohort study on height and risk of cancer and cancer death. Cancer Causes Control CCC 25:151–159. 10.1007/s10552-013-0317-7 [ DOI ] [ PMC free article ] [ PubMed ] [ Google Scholar ]
- 75. Zhang B, Shu X-O, Delahanty RJ, et al. (2015) Height and Breast Cancer Risk: Evidence From Prospective Studies and Mendelian Randomization. J Natl Cancer Inst 107:. 10.1093/jnci/djv219 [ DOI ] [ PMC free article ] [ PubMed ] [ Google Scholar ]
- 76. Xue F, Michels KB (2007) Intrauterine factors and risk of breast cancer: a systematic review and meta-analysis of current evidence. Lancet Oncol 8:1088–1100. 10.1016/S1470-2045(07)70377-7 [ DOI ] [ PubMed ] [ Google Scholar ]
- 77. Bertrand KA, Scott CG, Tamimi RM, et al. (2015) Dense and nondense mammographic area and risk of breast cancer by age and tumor characteristics. Cancer Epidemiol Biomark Prev Publ Am Assoc Cancer Res Cosponsored Am Soc Prev Oncol 24:798–809. 10.1158/1055-9965.EPI-14-1136 [ DOI ] [ PMC free article ] [ PubMed ] [ Google Scholar ]
- 78. Pettersson A, Graff RE, Ursin G, et al. (2014) Mammographic density phenotypes and risk of breast cancer: a meta-analysis. J Natl Cancer Inst 106:. 10.1093/jnci/dju078 [ DOI ] [ PMC free article ] [ PubMed ] [ Google Scholar ]
- 79. Bae J-M, Kim EH (2016) Breast Density and Risk of Breast Cancer in Asian Women: A Meta-analysis of Observational Studies. J Prev Med Public Health Yebang Uihakhoe Chi 49:367–375. 10.3961/jpmph.16.054 [ DOI ] [ PMC free article ] [ PubMed ] [ Google Scholar ]
- 80. Kyanko KA, Hoag J, Busch SH, et al. (2020) Dense Breast Notification Laws, Education, and Women’s Awareness and Knowledge of Breast Density: a Nationally Representative Survey. J Gen Intern Med. 10.1007/s11606-019-05590-7 [ DOI ] [ PMC free article ] [ PubMed ] [ Google Scholar ]
- 81. Chen J-H, Yuan Q, Ma Y-N, et al. (2019) Relationship between bone mineral density and the risk of breast cancer: a systematic review and dose-response meta-analysis of ten cohort studies. Cancer Manag Res 11:1453–1464. 10.2147/CMAR.S188251 [ DOI ] [ PMC free article ] [ PubMed ] [ Google Scholar ]
- 82. Nagel G, Peter RS, Klotz E, et al. (2017) Bone mineral density and breast cancer risk: Results from the Vorarlberg Health Monitoring & Prevention Program and meta-analysis. Bone Rep 7:83–89. 10.1016/j.bonr.2017.09.004 [ DOI ] [ PMC free article ] [ PubMed ] [ Google Scholar ]
- 83. Collaborative Group on Hormonal Factors in Breast Cancer (2012) Menarche, menopause, and breast cancer risk: individual participant meta-analysis, including 118 964 women with breast cancer from 117 epidemiological studies. Lancet Oncol 13:1141–1151. 10.1016/S1470-2045(12)70425-4 [ DOI ] [ PMC free article ] [ PubMed ] [ Google Scholar ]
- 84. Collaborative Group on Hormonal Factors in Breast Cancer (2002) Breast cancer and breastfeeding: collaborative reanalysis of individual data from 47 epidemiological studies in 30 countries, including 50302 women with breast cancer and 96973 women without the disease. Lancet Lond Engl 360:187–195. 10.1016/S0140-6736(02)09454-0 [ DOI ] [ PubMed ] [ Google Scholar ]
- 85. Nichols HB, Schoemaker MJ, Cai J, et al. (2019) Breast Cancer Risk After Recent Childbirth: A Pooled Analysis of 15 Prospective Studies. Ann Intern Med 170:22–30. 10.7326/M18-1323 [ DOI ] [ PMC free article ] [ PubMed ] [ Google Scholar ]
- 86. Lambertini M, Santoro L, Del Mastro L, et al. (2016) Reproductive behaviors and risk of developing breast cancer according to tumor subtype: A systematic review and meta-analysis of epidemiological studies. Cancer Treat Rev 49:65–76. 10.1016/j.ctrv.2016.07.006 [ DOI ] [ PubMed ] [ Google Scholar ]
- 87. Ma H, Bernstein L, Pike MC, Ursin G (2006) Reproductive factors and breast cancer risk according to joint estrogen and progesterone receptor status: a meta-analysis of epidemiological studies. Breast Cancer Res BCR 8:R43. 10.1186/bcr1525 [ DOI ] [ PMC free article ] [ PubMed ] [ Google Scholar ]
- 88. MacMahon B, Cole P, Lin TM, et al. (1970) Age at first birth and breast cancer risk. Bull World Health Organ 43:209–221 [ PMC free article ] [ PubMed ] [ Google Scholar ]
- 89. Mallick S, Benson R, Julka PK (2016) Breast cancer prevention with anti-estrogens: review of the current evidence and future directions. Breast Cancer Tokyo Jpn 23:170–177. 10.1007/s12282-015-0647-2 [ DOI ] [ PubMed ] [ Google Scholar ]
- 90. Samavat H, Kurzer MS (2015) Estrogen metabolism and breast cancer. Cancer Lett 356:231–243. 10.1016/j.canlet.2014.04.018 [ DOI ] [ PMC free article ] [ PubMed ] [ Google Scholar ]
- 91. Endogenous Hormones and Breast Cancer Collaborative Group, Key TJ, Appleby PN, et al. (2013) Sex hormones and risk of breast cancer in premenopausal women: a collaborative reanalysis of individual participant data from seven prospective studies. Lancet Oncol 14:1009–1019. 10.1016/S1470-2045(13)70301-2 [ DOI ] [ PMC free article ] [ PubMed ] [ Google Scholar ]
- 92. (2002) Endogenous Sex Hormones and Breast Cancer in Postmenopausal Women: Reanalysis of Nine Prospective Studies. JNCI J Natl Cancer Inst 94:606–616. 10.1093/jnci/94.8.606 [ DOI ] [ PubMed ] [ Google Scholar ]
- 93. Key TJ, Appleby PN, Reeves GK, et al. (2015) Steroid hormone measurements from different types of assays in relation to body mass index and breast cancer risk in postmenopausal women: Reanalysis of eighteen prospective studies. Steroids 99:49–55. 10.1016/j.steroids.2014.09.001 [ DOI ] [ PMC free article ] [ PubMed ] [ Google Scholar ]
- 94. Wang M, Wu X, Chai F, et al. (2016) Plasma prolactin and breast cancer risk: a meta- analysis. Sci Rep 6:25998. 10.1038/srep25998 [ DOI ] [ PMC free article ] [ PubMed ] [ Google Scholar ]
- 95. Ziegler RG, Fuhrman BJ, Moore SC, Matthews CE (2015) Epidemiologic studies of estrogen metabolism and breast cancer. Steroids 99:67–75. 10.1016/j.steroids.2015.02.015 [ DOI ] [ PMC free article ] [ PubMed ] [ Google Scholar ]
- 96. Sampson JN, Falk RT, Schairer C, et al. (2017) Association of Estrogen Metabolism with Breast Cancer Risk in Different Cohorts of Postmenopausal Women. Cancer Res 77:918–925. 10.1158/0008-5472.CAN-16-1717 [ DOI ] [ PMC free article ] [ PubMed ] [ Google Scholar ]
- 97. Dallal CM, Stone RA, Cauley JA, et al. (2013) Urinary estrogen metabolites and breast cancer: a combined analysis of individual level data. Int J Biol Markers 28:3–16. 10.5301/JBM.2012.9353 [ DOI ] [ PubMed ] [ Google Scholar ]
- 98. Ge W, Clendenen TV, Afanasyeva Y, et al. (2018) Circulating anti-Müllerian hormone and breast cancer risk: A study in ten prospective cohorts. Int J Cancer 142:2215–2226. 10.1002/ijc.31249 [ DOI ] [ PMC free article ] [ PubMed ] [ Google Scholar ]
- 99. He XY, Liao YD, Yu S, et al. (2015) Sex hormone binding globulin and risk of breast cancer in postmenopausal women: a meta-analysis of prospective studies. Horm Metab Res Horm Stoffwechselforschung Horm Metab 47:485–490. 10.1055/s-0034-1395606 [ DOI ] [ PubMed ] [ Google Scholar ]
- 100. Missmer SA, Eliassen AH, Barbieri RL, Hankinson SE (2004) Endogenous estrogen, androgen, and progesterone concentrations and breast cancer risk among postmenopausal women. J Natl Cancer Inst 96:1856–1865. 10.1093/jnci/djh336 [ DOI ] [ PubMed ] [ Google Scholar ]
- 101. Endogenous Hormones and Breast Cancer Collaborative Group, Key TJ, Appleby PN, et al. (2010) Insulin-like growth factor 1 (IGF1), IGF binding protein 3 (IGFBP3), and breast cancer risk: pooled individual data analysis of 17 prospective studies. Lancet Oncol 11:530–542. 10.1016/S1470-2045(10)70095-4 [ DOI ] [ PMC free article ] [ PubMed ] [ Google Scholar ]
- 102. Murphy N, Knuppel A, Papadimitriou N, et al. (2020) Insulin-like growth factor-1, insulin-like growth factor-binding protein-3, and breast cancer risk: observational and Mendelian randomization analyses with ~430 000 women. Ann Oncol 31:641–649. 10.1016/j.annonc.2020.01.066 [ DOI ] [ PMC free article ] [ PubMed ] [ Google Scholar ]
- 103. Autier P, Koechlin A, Boniol M, et al. (2013) Serum insulin and C-peptide concentration and breast cancer: a meta-analysis. Cancer Causes Control CCC 24:873–883. 10.1007/s10552-013-0164-6 [ DOI ] [ PubMed ] [ Google Scholar ]
- 104. Hernandez AV, Guarnizo M, Miranda Y, et al. (2014) Association between insulin resistance and breast carcinoma: a systematic review and meta-analysis. PloS One 9:e99317. 10.1371/journal.pone.0099317 [ DOI ] [ PMC free article ] [ PubMed ] [ Google Scholar ]
- 105. Shen J, Hernandez D, Ye Y, et al. (2019) Metabolic hormones and breast cancer risk among Mexican American Women in the Mano a Mano Cohort Study. Sci Rep 9:9989. 10.1038/s41598-019-46429-9 [ DOI ] [ PMC free article ] [ PubMed ] [ Google Scholar ]
- 106. Ahern TP, Hankinson SE, Willett WC, et al. (2013) Plasma C-peptide, mammographic breast density, and risk of invasive breast cancer. Cancer Epidemiol Biomark Prev Publ Am Assoc Cancer Res Cosponsored Am Soc Prev Oncol 22:1786–1796. 10.1158/1055-9965.EPI-13-0375 [ DOI ] [ PMC free article ] [ PubMed ] [ Google Scholar ]
- 107. Gaudet MM, Patel AV, Teras LR, et al. (2013) Obesity-related markers and breast cancer in CPS-II Nutrition Cohort. Int J Mol Epidemiol Genet 4:156–166 [ PMC free article ] [ PubMed ] [ Google Scholar ]
- 108. Wang J, Lee I-M, Tworoger SS, et al. (2015) Plasma C-reactive protein and risk of breast cancer in two prospective studies and a meta-analysis. Cancer Epidemiol Biomark Prev Publ Am Assoc Cancer Res Cosponsored Am Soc Prev Oncol 24:1199–1206. 10.1158/1055-9965.EPI-15-0187 [ DOI ] [ PMC free article ] [ PubMed ] [ Google Scholar ]
- 109. Chan DSM, Bandera EV, Greenwood DC, Norat T (2015) Circulating C-Reactive Protein and Breast Cancer Risk-Systematic Literature Review and Meta-analysis of Prospective Cohort Studies. Cancer Epidemiol Biomark Prev Publ Am Assoc Cancer Res Cosponsored Am Soc Prev Oncol 24:1439–1449. 10.1158/1055-9965.EPI-15-0324 [ DOI ] [ PubMed ] [ Google Scholar ]
- 110. Guo L, Liu S, Zhang S, et al. (2015) C-reactive protein and risk of breast cancer: A systematic review and meta-analysis. Sci Rep 5:10508. 10.1038/srep10508 [ DOI ] [ PMC free article ] [ PubMed ] [ Google Scholar ]
- 111. Pan H, Deng L-L, Cui J-Q, et al. (2018) Association between serum leptin levels and breast cancer risk: An updated systematic review and meta-analysis. Medicine (Baltimore) 97:e11345. 10.1097/MD.0000000000011345 [ DOI ] [ PMC free article ] [ PubMed ] [ Google Scholar ]
- 112. Yu Z, Tang S, Ma H, et al. (2019) Association of serum adiponectin with breast cancer: A meta-analysis of 27 case-control studies. Medicine (Baltimore) 98:e14359. 10.1097/MD.0000000000014359 [ DOI ] [ PMC free article ] [ PubMed ] [ Google Scholar ]
- 113. Xu J, Huang L, Sun G-P (2017) Urinary 6-sulfatoxymelatonin level and breast cancer risk: systematic review and meta-analysis. Sci Rep 7:5353. 10.1038/s41598-017-05752-9 [ DOI ] [ PMC free article ] [ PubMed ] [ Google Scholar ]
- 114. Veiga EC de A, Simões R, Valenti VE, et al. (2019) Repercussions of melatonin on the risk of breast cancer: a systematic review and meta-analysis. Rev Assoc Medica Bras 1992 65:699–705. 10.1590/1806-9282.65.5.699 [ DOI ] [ PubMed ] [ Google Scholar ]
- 115. Ji L-W, Jing C-X, Zhuang S-L, et al. (2019) Effect of age at first use of oral contraceptives on breast cancer risk: An updated meta-analysis. Medicine (Baltimore) 98:e15719. 10.1097/MD.0000000000015719 [ DOI ] [ PMC free article ] [ PubMed ] [ Google Scholar ]
- 116. Zhu H, Lei X, Feng J, Wang Y (2012) Oral contraceptive use and risk of breast cancer: a meta-analysis of prospective cohort studies. Eur J Contracept Reprod Health Care Off J Eur Soc Contracept 17:402–414. 10.3109/13625187.2012.715357 [ DOI ] [ PubMed ] [ Google Scholar ]
- 117. (1996) Breast cancer and hormonal contraceptives: further results. Collaborative Group on Hormonal Factors in Breast Cancer. Contraception 54:1S–106S. 10.1016/s0010-7824(15)30002-0 [ DOI ] [ PubMed ] [ Google Scholar ]
- 118. Conz L, Mota BS, Bahamondes L, et al. (2020) Levonorgestrel-releasing intrauterine system and breast cancer risk: A systematic review and meta-analysis. Acta Obstet Gynecol Scand. 10.1111/aogs.13817 [ DOI ] [ PubMed ] [ Google Scholar ]
- 119. Collaborative Group on Hormonal Factors in Breast Cancer (2019) Type and timing of menopausal hormone therapy and breast cancer risk: individual participant meta-analysis of the worldwide epidemiological evidence. Lancet Lond Engl 394:1159–1168. 10.1016/S0140-6736(19)31709-X [ DOI ] [ PMC free article ] [ PubMed ] [ Google Scholar ]
- 120. Jordan VC (2015) The new biology of estrogen-induced apoptosis applied to treat and prevent breast cancer. Endocr Relat Cancer 22:R1–31. 10.1530/ERC-14-0448 [ DOI ] [ PMC free article ] [ PubMed ] [ Google Scholar ]
- 121. Key TJ, Appleby PN, Cairns BJ, et al. (2011) Dietary fat and breast cancer: comparison of results from food diaries and food-frequency questionnaires in the UK Dietary Cohort Consortium. Am J Clin Nutr 94:1043–1052. 10.3945/ajcn.111.015735 [ DOI ] [ PubMed ] [ Google Scholar ]
- 122. Smith-Warner SA, Spiegelman D, Adami HO, et al. (2001) Types of dietary fat and breast cancer: a pooled analysis of cohort studies. Int J Cancer 92:767–774. [ DOI ] [ PubMed ] [ Google Scholar ]
- 123. Anjom-Shoae J, Sadeghi O, Larijani B, Esmaillzadeh A (2020) Dietary intake and serum levels of trans fatty acids and risk of breast cancer: A systematic review and dose-response meta-analysis of prospective studies. Clin Nutr Edinb Scotl 39:755–764. 10.1016/j.clnu.2019.03.024 [ DOI ] [ PubMed ] [ Google Scholar ]
- 124. Cao Y, Hou L, Wang W (2016) Dietary total fat and fatty acids intake, serum fatty acids and risk of breast cancer: A meta-analysis of prospective cohort studies. Int J Cancer 138:1894–1904. 10.1002/ijc.29938 [ DOI ] [ PubMed ] [ Google Scholar ]
- 125. Alexander DD, Morimoto LM, Mink PJ, Lowe KA (2010) Summary and meta-analysis of prospective studies of animal fat intake and breast cancer. Nutr Res Rev 23:169–179. 10.1017/S095442241000003X [ DOI ] [ PubMed ] [ Google Scholar ]
- 126. Hunter DJ, Spiegelman D, Adami HO, et al. (1996) Cohort studies of fat intake and the risk of breast cancer--a pooled analysis. N Engl J Med 334:356–361. 10.1056/NEJM199602083340603 [ DOI ] [ PubMed ] [ Google Scholar ]
- 127. Schlesinger S, Chan DSM, Vingeliene S, et al. (2017) Carbohydrates, glycemic index, glycemic load, and breast cancer risk: a systematic review and dose-response meta-analysis of prospective studies. Nutr Rev 75:420–441. 10.1093/nutrit/nux010 [ DOI ] [ PubMed ] [ Google Scholar ]
- 128. Mullie P, Koechlin A, Boniol M, et al. (2016) Relation between Breast Cancer and High Glycemic Index or Glycemic Load: A Meta-analysis of Prospective Cohort Studies. Crit Rev Food Sci Nutr 56:152–159. 10.1080/10408398.2012.718723 [ DOI ] [ PubMed ] [ Google Scholar ]
- 129. Chen S, Chen Y, Ma S, et al. (2016) Dietary fibre intake and risk of breast cancer: A systematic review and meta-analysis of epidemiological studies. Oncotarget 7:80980–80989. 10.18632/oncotarget.13140 [ DOI ] [ PMC free article ] [ PubMed ] [ Google Scholar ]
- 130. Aune D, Chan DSM, Greenwood DC, et al. (2012) Dietary fiber and breast cancer risk: a systematic review and meta-analysis of prospective studies. Ann Oncol Off J Eur Soc Med Oncol 23:1394–1402. 10.1093/annonc/mdr589 [ DOI ] [ PubMed ] [ Google Scholar ]
- 131. Dong J-Y, He K, Wang P, Qin L-Q (2011) Dietary fiber intake and risk of breast cancer: a meta-analysis of prospective cohort studies. Am J Clin Nutr 94:900–905. 10.3945/ajcn.111.015578 [ DOI ] [ PubMed ] [ Google Scholar ]
- 132. Farvid MS, Stern MC, Norat T, et al. (2018) Consumption of red and processed meat and breast cancer incidence: A systematic review and meta-analysis of prospective studies. Int J Cancer 143:2787–2799. 10.1002/ijc.31848 [ DOI ] [ PMC free article ] [ PubMed ] [ Google Scholar ]
- 133. Anderson JJ, Darwis NDM, Mackay DF, et al. (2018) Red and processed meat consumption and breast cancer: UK Biobank cohort study and meta-analysis. Eur J Cancer Oxf Engl 1990 90:73–82. 10.1016/j.ejca.2017.11.022 [ DOI ] [ PubMed ] [ Google Scholar ]
- 134. Wu J, Zeng R, Huang J, et al. (2016) Dietary Protein Sources and Incidence of Breast Cancer: A Dose-Response Meta-Analysis of Prospective Studies. Nutrients 8:. 10.3390/nu8110730 [ DOI ] [ PMC free article ] [ PubMed ] [ Google Scholar ]
- 135. Han MA, Zeraatkar D, Guyatt GH, et al. (2019) Reduction of Red and Processed Meat Intake and Cancer Mortality and Incidence: A Systematic Review and Meta-analysis of Cohort Studies. Ann Intern Med 171:711–720. 10.7326/M19-0699 [ DOI ] [ PubMed ] [ Google Scholar ]
- 136. Missmer SA, Smith-Warner SA, Spiegelman D, et al. (2002) Meat and dairy food consumption and breast cancer: a pooled analysis of cohort studies. Int J Epidemiol 31:78–85. 10.1093/ije/31.1.78 [ DOI ] [ PubMed ] [ Google Scholar ]
- 137. Dong J-Y, Zhang L, He K, Qin L-Q (2011) Dairy consumption and risk of breast cancer: a meta-analysis of prospective cohort studies. Breast Cancer Res Treat 127:23–31. 10.1007/s10549-011-1467-5 [ DOI ] [ PubMed ] [ Google Scholar ]
- 138. Chen L, Li M, Li H (2019) Milk and yogurt intake and breast cancer risk: A meta-analysis. Medicine (Baltimore) 98:e14900. 10.1097/MD.0000000000014900 [ DOI ] [ PMC free article ] [ PubMed ] [ Google Scholar ]
- 139. Jung S, Spiegelman D, Baglietto L, et al. (2013) Fruit and vegetable intake and risk of breast cancer by hormone receptor status. J Natl Cancer Inst 105:219–236. 10.1093/jnci/djs635 [ DOI ] [ PMC free article ] [ PubMed ] [ Google Scholar ]
- 140. Aune D, Chan DSM, Vieira AR, et al. (2012) Fruits, vegetables and breast cancer risk: a systematic review and meta-analysis of prospective studies. Breast Cancer Res Treat 134:479–493. 10.1007/s10549-012-2118-1 [ DOI ] [ PubMed ] [ Google Scholar ]
- 141. Smith-Warner SA, Spiegelman D, Yaun SS, et al. (2001) Intake of fruits and vegetables and risk of breast cancer: a pooled analysis of cohort studies. JAMA 285:769–776. 10.1001/jama.285.6.769 [ DOI ] [ PubMed ] [ Google Scholar ]
- 142. Jiang W, Wu Y, Jiang X (2013) Coffee and caffeine intake and breast cancer risk: an updated dose-response meta-analysis of 37 published studies. Gynecol Oncol 129:620–629. 10.1016/j.ygyno.2013.03.014 [ DOI ] [ PubMed ] [ Google Scholar ]
- 143. Lafranconi A, Micek A, De Paoli P, et al. (2018) Coffee Intake Decreases Risk of Postmenopausal Breast Cancer: A Dose-Response Meta-Analysis on Prospective Cohort Studies. Nutrients 10:. 10.3390/nu10020112 [ DOI ] [ PMC free article ] [ PubMed ] [ Google Scholar ]
- 144. Wang Y, Zhao Y, Chong F, et al. (2020) A dose-response meta-analysis of green tea consumption and breast cancer risk. Int J Food Sci Nutr 1–12. 10.1080/09637486.2020.1715353 [ DOI ] [ PubMed ] [ Google Scholar ]
- 145. Bhoo-Pathy N, Peeters PHM, Uiterwaal CSPM, et al. (2015) Coffee and tea consumption and risk of pre- and postmenopausal breast cancer in the European Prospective Investigation into Cancer and Nutrition (EPIC) cohort study. Breast Cancer Res BCR 17:15. 10.1186/s13058-015-0521-3 [ DOI ] [ PMC free article ] [ PubMed ] [ Google Scholar ]
- 146. Yu S, Zhu L, Wang K, et al. (2019) Green tea consumption and risk of breast cancer: A systematic review and updated meta-analysis of case-control studies. Medicine (Baltimore) 98:e16147. 10.1097/MD.0000000000016147 [ DOI ] [ PMC free article ] [ PubMed ] [ Google Scholar ]
- 147. Bristow SM, Bolland MJ, MacLennan GS, et al. (2013) Calcium supplements and cancer risk: a meta-analysis of randomised controlled trials. Br J Nutr 110:1384–1393. 10.1017/S0007114513001050 [ DOI ] [ PubMed ] [ Google Scholar ]
- 148. Hidayat K, Chen G-C, Zhang R, et al. (2016) Calcium intake and breast cancer risk: meta-analysis of prospective cohort studies. Br J Nutr 116:158–166. 10.1017/S0007114516001768 [ DOI ] [ PubMed ] [ Google Scholar ]
- 149. Wulaningsih W, Sagoo HK, Hamza M, et al. (2016) Serum Calcium and the Risk of Breast Cancer: Findings from the Swedish AMORIS Study and a Meta-Analysis of Prospective Studies. Int J Mol Sci 17:. 10.3390/ijms17091487 [ DOI ] [ PMC free article ] [ PubMed ] [ Google Scholar ]
- 150. Estébanez N, Gómez-Acebo I, Palazuelos C, et al. (2018) Vitamin D exposure and Risk of Breast Cancer: a meta-analysis. Sci Rep 8:9039. 10.1038/s41598-018-27297-1 [ DOI ] [ PMC free article ] [ PubMed ] [ Google Scholar ]
- 151. Sperati F, Vici P, Maugeri-Saccà M, et al. (2013) Vitamin D supplementation and breast cancer prevention: a systematic review and meta-analysis of randomized clinical trials. PloS One 8:e69269. 10.1371/journal.pone.0069269 [ DOI ] [ PMC free article ] [ PubMed ] [ Google Scholar ]
- 152. Hossain S, Beydoun MA, Beydoun HA, et al. (2019) Vitamin D and breast cancer: A systematic review and meta-analysis of observational studies. Clin Nutr ESPEN 30:170–184. 10.1016/j.clnesp.2018.12.085 [ DOI ] [ PMC free article ] [ PubMed ] [ Google Scholar ]
- 153. Song D, Deng Y, Liu K, et al. (2019) Vitamin D intake, blood vitamin D levels, and the risk of breast cancer: a dose-response meta-analysis of observational studies. Aging 11:12708–12732. 10.18632/aging.102597 [ DOI ] [ PMC free article ] [ PubMed ] [ Google Scholar ]
- 154. Eliassen AH, Hendrickson SJ, Brinton LA, et al. (2012) Circulating carotenoids and risk of breast cancer: pooled analysis of eight prospective studies. J Natl Cancer Inst 104:1905–1916. 10.1093/jnci/djs461 [ DOI ] [ PMC free article ] [ PubMed ] [ Google Scholar ]
- 155. Zhang X, Spiegelman D, Baglietto L, et al. (2012) Carotenoid intakes and risk of breast cancer defined by estrogen receptor and progesterone receptor status: a pooled analysis of 18 prospective cohort studies. Am J Clin Nutr 95:713–725. 10.3945/ajcn.111.014415 [ DOI ] [ PMC free article ] [ PubMed ] [ Google Scholar ]
- 156. Aune D, Chan DSM, Vieira AR, et al. (2012) Dietary compared with blood concentrations of carotenoids and breast cancer risk: a systematic review and meta-analysis of prospective studies. Am J Clin Nutr 96:356–373. 10.3945/ajcn.112.034165 [ DOI ] [ PubMed ] [ Google Scholar ]
- 157. Druesne-Pecollo N, Latino-Martel P, Norat T, et al. (2010) Beta-carotene supplementation and cancer risk: a systematic review and metaanalysis of randomized controlled trials. Int J Cancer 127:172–184. 10.1002/ijc.25008 [ DOI ] [ PubMed ] [ Google Scholar ]
- 158. Chang VC, Cotterchio M, Khoo E (2019) Iron intake, body iron status, and risk of breast cancer: a systematic review and meta-analysis. BMC Cancer 19:543. 10.1186/s12885-019-5642-0 [ DOI ] [ PMC free article ] [ PubMed ] [ Google Scholar ]
- 159. Fulan H, Changxing J, Baina WY, et al. (2011) Retinol, vitamins A, C, and E and breast cancer risk: a meta-analysis and meta-regression. Cancer Causes Control CCC 22:1383–1396. 10.1007/s10552-011-9811-y [ DOI ] [ PubMed ] [ Google Scholar ]
- 160. Wu W, Kang S, Zhang D (2013) Association of vitamin B6, vitamin B12 and methionine with risk of breast cancer: a dose-response meta-analysis. Br J Cancer 109:1926–1944. 10.1038/bjc.2013.438 [ DOI ] [ PMC free article ] [ PubMed ] [ Google Scholar ]
- 161. Yu L, Tan Y, Zhu L (2017) Dietary vitamin B2 intake and breast cancer risk: a systematic review and meta-analysis. Arch Gynecol Obstet 295:721–729. 10.1007/s00404-016-4278-4 [ DOI ] [ PubMed ] [ Google Scholar ]
- 162. Hu F, Wu Z, Li G, et al. (2015) The plasma level of retinol, vitamins A, C and α-tocopherol could reduce breast cancer risk? A meta-analysis and meta-regression. J Cancer Res Clin Oncol 141:601–614. 10.1007/s00432-014-1852-7 [ DOI ] [ PubMed ] [ Google Scholar ]
- 163. Hutchinson J, Lentjes M a. H, Greenwood DC, et al. (2012) Vitamin C intake from diary recordings and risk of breast cancer in the UK Dietary Cohort Consortium. Eur J Clin Nutr 66:561–568. 10.1038/ejcn.2011.197 [ DOI ] [ PMC free article ] [ PubMed ] [ Google Scholar ]
- 164. Chen P, Li C, Li X, et al. (2014) Higher dietary folate intake reduces the breast cancer risk: a systematic review and meta-analysis. Br J Cancer 110:2327–2338. 10.1038/bjc.2014.155 [ DOI ] [ PMC free article ] [ PubMed ] [ Google Scholar ]
- 165. Zhang Y-F, Shi W-W, Gao H-F, et al. (2014) Folate intake and the risk of breast cancer: a dose-response meta-analysis of prospective studies. PloS One 9:e100044. 10.1371/journal.pone.0100044 [ DOI ] [ PMC free article ] [ PubMed ] [ Google Scholar ]
- 166. Qin X, Cui Y, Shen L, et al. (2013) Folic acid supplementation and cancer risk: a meta-analysis of randomized controlled trials. Int J Cancer 133:1033–1041. 10.1002/ijc.28038 [ DOI ] [ PubMed ] [ Google Scholar ]
- 167. Tio M, Andrici J, Eslick GD (2014) Folate intake and the risk of breast cancer: a systematic review and meta-analysis. Breast Cancer Res Treat 145:513–524. 10.1007/s10549-014-2969-8 [ DOI ] [ PubMed ] [ Google Scholar ]
- 168. Zeng J, Wang K, Ye F, et al. (2019) Folate intake and the risk of breast cancer: an up-to-date meta-analysis of prospective studies. Eur J Clin Nutr 73:1657–1660. 10.1038/s41430-019-0394-0 [ DOI ] [ PubMed ] [ Google Scholar ]
- 169. Cai X, Wang C, Yu W, et al. (2016) Selenium Exposure and Cancer Risk: an Updated Meta-analysis and Meta-regression. Sci Rep 6:19213. 10.1038/srep19213 [ DOI ] [ PMC free article ] [ PubMed ] [ Google Scholar ]
- 170. Babaknejad N, Sayehmiri F, Sayehmiri K, et al. (2014) The relationship between selenium levels and breast cancer: a systematic review and meta-analysis. Biol Trace Elem Res 159:1–7. 10.1007/s12011-014-9998-3 [ DOI ] [ PubMed ] [ Google Scholar ]
- 171. Velentzis LS, Cantwell MM, Cardwell C, et al. (2009) Lignans and breast cancer risk in pre- and post-menopausal women: meta-analyses of observational studies. Br J Cancer 100:1492–1498. 10.1038/sj.bjc.6605003 [ DOI ] [ PMC free article ] [ PubMed ] [ Google Scholar ]
- 172. Buck K, Zaineddin AK, Vrieling A, et al. (2010) Meta-analyses of lignans and enterolignans in relation to breast cancer risk. Am J Clin Nutr 92:141–153. 10.3945/ajcn.2009.28573 [ DOI ] [ PubMed ] [ Google Scholar ]
- 173. Chen M, Rao Y, Zheng Y, et al. (2014) Association between soy isoflavone intake and breast cancer risk for pre- and post-menopausal women: a meta-analysis of epidemiological studies. PloS One 9:e89288. 10.1371/journal.pone.0089288 [ DOI ] [ PMC free article ] [ PubMed ] [ Google Scholar ]
- 174. Zhao T-T, Jin F, Li J-G, et al. (2019) Dietary isoflavones or isoflavone-rich food intake and breast cancer risk: A meta-analysis of prospective cohort studies. Clin Nutr Edinb Scotl 38:136–145. 10.1016/j.clnu.2017.12.006 [ DOI ] [ PubMed ] [ Google Scholar ]
- 175. Wei Y, Lv J, Guo Y, et al. (2020) Soy intake and breast cancer risk: a prospective study of 300,000 Chinese women and a dose-response meta-analysis. Eur J Epidemiol 35:567–578. 10.1007/s10654-019-00585-4 [ DOI ] [ PMC free article ] [ PubMed ] [ Google Scholar ]
- 176. Wang Q, Liu X, Ren S (2020) Tofu intake is inversely associated with risk of breast cancer: A meta-analysis of observational studies. PloS One 15:e0226745. 10.1371/journal.pone.0226745 [ DOI ] [ PMC free article ] [ PubMed ] [ Google Scholar ]
- 177. Chan ALF, Leung HWC, Wang S-F (2011) Multivitamin supplement use and risk of breast cancer: a meta-analysis. Ann Pharmacother 45:476–484. 10.1345/aph.1P445 [ DOI ] [ PubMed ] [ Google Scholar ]
- 178. Smith-Warner SA, Spiegelman D, Yaun SS, et al. (1998) Alcohol and breast cancer in women: a pooled analysis of cohort studies. JAMA 279:535–540. 10.1001/jama.279.7.535 [ DOI ] [ PubMed ] [ Google Scholar ]
- 179. Bagnardi V, Rota M, Botteri E, et al. (2015) Alcohol consumption and site-specific cancer risk: a comprehensive dose-response meta-analysis. Br J Cancer 112:580–593. 10.1038/bjc.2014.579 [ DOI ] [ PMC free article ] [ PubMed ] [ Google Scholar ]
- 180. Jung S, Wang M, Anderson K, et al. (2016) Alcohol consumption and breast cancer risk by estrogen receptor status: in a pooled analysis of 20 studies. Int J Epidemiol 45:916–928. 10.1093/ije/dyv156 [ DOI ] [ PMC free article ] [ PubMed ] [ Google Scholar ]
- 181. Liu Y, Nguyen N, Colditz GA (2015) Links between alcohol consumption and breast cancer: a look at the evidence. Womens Health Lond Engl 11:65–77. 10.2217/whe.14.62 [ DOI ] [ PMC free article ] [ PubMed ] [ Google Scholar ]
- 182. Hamajima N, Hirose K, Tajima K, et al. (2002) Alcohol, tobacco and breast cancer--collaborative reanalysis of individual data from 53 epidemiological studies, including 58,515 women with breast cancer and 95,067 women without the disease. Br J Cancer 87:1234–1245. 10.1038/sj.bjc.6600596 [ DOI ] [ PMC free article ] [ PubMed ] [ Google Scholar ]
- 183. Keogh RH, Park JY, White IR, et al. (2012) Estimating the alcohol-breast cancer association: a comparison of diet diaries, FFQs and combined measurements. Eur J Epidemiol 27:547–559. 10.1007/s10654-012-9693-7 [ DOI ] [ PubMed ] [ Google Scholar ]
- 184. Schwingshackl L, Schwedhelm C, Galbete C, Hoffmann G (2017) Adherence to Mediterranean Diet and Risk of Cancer: An Updated Systematic Review and Meta-Analysis. Nutrients 9:. 10.3390/nu9101063 [ DOI ] [ PMC free article ] [ PubMed ] [ Google Scholar ]
- 185. van den Brandt PA, Schulpen M (2017) Mediterranean diet adherence and risk of postmenopausal breast cancer: results of a cohort study and meta-analysis. Int J Cancer 140:2220–2231. 10.1002/ijc.30654 [ DOI ] [ PubMed ] [ Google Scholar ]
- 186. Grosso G, Bella F, Godos J, et al. (2017) Possible role of diet in cancer: systematic review and multiple meta-analyses of dietary patterns, lifestyle factors, and cancer risk. Nutr Rev 75:405–419. 10.1093/nutrit/nux012 [ DOI ] [ PubMed ] [ Google Scholar ]
- 187. Xiao Y, Xia J, Li L, et al. (2019) Associations between dietary patterns and the risk of breast cancer: a systematic review and meta-analysis of observational studies. Breast Cancer Res BCR 21:16. 10.1186/s13058-019-1096-1 [ DOI ] [ PMC free article ] [ PubMed ] [ Google Scholar ]
- 188. Pot GK, Stephen AM, Dahm CC, et al. (2014) Dietary patterns derived with multiple methods from food diaries and breast cancer risk in the UK Dietary Cohort Consortium. Eur J Clin Nutr 68:1353–1358. 10.1038/ejcn.2014.135 [ DOI ] [ PMC free article ] [ PubMed ] [ Google Scholar ]
- 189. Toledo E, Salas-Salvadó J, Donat-Vargas C, et al. (2015) Mediterranean Diet and Invasive Breast Cancer Risk Among Women at High Cardiovascular Risk in the PREDIMED Trial: A Randomized Clinical Trial. JAMA Intern Med 175:1752–1760. 10.1001/jamainternmed.2015.4838 [ DOI ] [ PubMed ] [ Google Scholar ]
- 190. Macacu A, Autier P, Boniol M, Boyle P (2015) Active and passive smoking and risk of breast cancer: a meta-analysis. Breast Cancer Res Treat 154:213–224. 10.1007/s10549-015-3628-4 [ DOI ] [ PubMed ] [ Google Scholar ]
- 191. Lee PN, Hamling JS (2016) Environmental tobacco smoke exposure and risk of breast cancer in nonsmoking women. An updated review and meta-analysis. Inhal Toxicol 28:431–454. 10.1080/08958378.2016.1210701 [ DOI ] [ PMC free article ] [ PubMed ] [ Google Scholar ]
- 192. Kim A-S, Ko H-J, Kwon J-H, Lee J-M (2018) Exposure to Secondhand Smoke and Risk of Cancer in Never Smokers: A Meta-Analysis of Epidemiologic Studies. Int J Environ Res Public Health 15:. 10.3390/ijerph15091981 [ DOI ] [ PMC free article ] [ PubMed ] [ Google Scholar ]
- 193. Yang Y, Zhang F, Skrip L, et al. (2013) Lack of an association between passive smoking and incidence of female breast cancer in non-smokers: evidence from 10 prospective cohort studies. PloS One 8:e77029. 10.1371/journal.pone.0077029 [ DOI ] [ PMC free article ] [ PubMed ] [ Google Scholar ]
- 194. Andersen ZJ, Stafoggia M, Weinmayr G, et al. (2017) Long-Term Exposure to Ambient Air Pollution and Incidence of Postmenopausal Breast Cancer in 15 European Cohorts within the ESCAPE Project. Environ Health Perspect 125:107005. 10.1289/EHP1742 [ DOI ] [ PMC free article ] [ PubMed ] [ Google Scholar ]
- 195. Zhang Z, Yan W, Chen Q, et al. (2019) The relationship between exposure to particulate matter and breast cancer incidence and mortality: A meta-analysis. Medicine (Baltimore) 98:e18349. 10.1097/MD.0000000000018349 [ DOI ] [ PMC free article ] [ PubMed ] [ Google Scholar ]
- 196. Chen Q, Lang L, Wu W, et al. (2013) A meta-analysis on the relationship between exposure to ELF-EMFs and the risk of female breast cancer. PloS One 8:e69272. 10.1371/journal.pone.0069272 [ DOI ] [ PMC free article ] [ PubMed ] [ Google Scholar ]
- 197. Zhao G, Lin X, Zhou M, Zhao J (2014) Relationship between exposure to extremely low-frequency electromagnetic fields and breast cancer risk: a meta-analysis. Eur J Gynaecol Oncol 35:264–269 [ PubMed ] [ Google Scholar ]
- 198. Zhang Y, Lai J, Ruan G, et al. (2016) Meta-analysis of extremely low frequency electromagnetic fields and cancer risk: a pooled analysis of epidemiologic studies. Environ Int 88:36–43. 10.1016/j.envint.2015.12.012 [ DOI ] [ PubMed ] [ Google Scholar ]
- 199. Guo J-Y, Wang M-Z, Wang M-S, et al. (2020) The Undervalued Effects of Polychlorinated Biphenyl Exposure on Breast Cancer. Clin Breast Cancer 20:12–18. 10.1016/j.clbc.2019.07.005 [ DOI ] [ PubMed ] [ Google Scholar ]
- 200. Zhang J, Huang Y, Wang X, et al. (2015) Environmental Polychlorinated Biphenyl Exposure and Breast Cancer Risk: A Meta-Analysis of Observational Studies. PloS One 10:e0142513. 10.1371/journal.pone.0142513 [ DOI ] [ PMC free article ] [ PubMed ] [ Google Scholar ]
- 201. Leng L, Li J, Luo X-M, et al. (2016) Polychlorinated biphenyls and breast cancer: A congener-specific meta-analysis. Environ Int 88:133–141. 10.1016/j.envint.2015.12.022 [ DOI ] [ PubMed ] [ Google Scholar ]
- 202. Ingber SZ, Buser MC, Pohl HR, et al. (2013) DDT/DDE and breast cancer: a meta-analysis. Regul Toxicol Pharmacol RTP 67:421–433. 10.1016/j.yrtph.2013.08.021 [ DOI ] [ PMC free article ] [ PubMed ] [ Google Scholar ]
- 203. Adani G, Filippini T, Wise LA, et al. (2020) Dietary Intake of Acrylamide and Risk of Breast, Endometrial, and Ovarian Cancers: A Systematic Review and Dose–Response Meta-analysis. Cancer Epidemiol Prev Biomark 29:1095–1106. 10.1158/1055-9965.EPI-19-1628 [ DOI ] [ PubMed ] [ Google Scholar ]
- 204. Barcellos-Hoff MH (2013) New biological insights on the link between radiation exposure and breast cancer risk. J Mammary Gland Biol Neoplasia 18:3–13. 10.1007/s10911-013-9272-x [ DOI ] [ PubMed ] [ Google Scholar ]
- 205. Preston DL, Mattsson A, Holmberg E, et al. (2002) Radiation effects on breast cancer risk: a pooled analysis of eight cohorts. Radiat Res 158:220–235. 10.1667/0033-7587(2002)158[0220:reobcr]2.0.co;2 [ DOI ] [ PubMed ] [ Google Scholar ]
- 206. Pizot C, Boniol M, Mullie P, et al. (2016) Physical activity, hormone replacement therapy and breast cancer risk: A meta-analysis of prospective studies. Eur J Cancer Oxf Engl 1990 52:138–154. 10.1016/j.ejca.2015.10.063 [ DOI ] [ PubMed ] [ Google Scholar ]
- 207. Neilson HK, Farris MS, Stone CR, et al. (2017) Moderate-vigorous recreational physical activity and breast cancer risk, stratified by menopause status: a systematic review and meta-analysis. Menopause N Y N 24:322–344. 10.1097/GME.0000000000000745 [ DOI ] [ PubMed ] [ Google Scholar ]
- 208. Chen X, Wang Q, Zhang Y, et al. (2019) Physical Activity and Risk of Breast Cancer: A Meta-Analysis of 38 Cohort Studies in 45 Study Reports. Value Health J Int Soc Pharmacoeconomics Outcomes Res 22:104–128. 10.1016/j.jval.2018.06.020 [ DOI ] [ PubMed ] [ Google Scholar ]
- 209. Gong Z, Hong C-C, Bandera EV, et al. (2016) Vigorous physical activity and risk of breast cancer in the African American breast cancer epidemiology and risk consortium. Breast Cancer Res Treat 159:347–356. 10.1007/s10549-016-3936-3 [ DOI ] [ PMC free article ] [ PubMed ] [ Google Scholar ]
- 210. Hidayat K, Zhou H-J, Shi B-M (2020) Influence of physical activity at a young age and lifetime physical activity on the risks of 3 obesity-related cancers: systematic review and meta-analysis of observational studies. Nutr Rev 78:1–18. 10.1093/nutrit/nuz024 [ DOI ] [ PubMed ] [ Google Scholar ]
- 211. Jochem C, Wallmann-Sperlich B, Leitzmann MF (2019) The Influence of Sedentary Behavior on Cancer Risk: Epidemiologic Evidence and Potential Molecular Mechanisms. Curr Nutr Rep 8:167–174. 10.1007/s13668-019-0263-4 [ DOI ] [ PubMed ] [ Google Scholar ]
- 212. Zhou Y, Zhao H, Peng C (2015) Association of sedentary behavior with the risk of breast cancer in women: update meta-analysis of observational studies. Ann Epidemiol 25:687–697. 10.1016/j.annepidem.2015.05.007 [ DOI ] [ PubMed ] [ Google Scholar ]
- 213. Gaudet MM, Carter BD, Brinton LA, et al. (2017) Pooled analysis of active cigarette smoking and invasive breast cancer risk in 14 cohort studies. Int J Epidemiol 46:881–893. 10.1093/ije/dyw288 [ DOI ] [ PMC free article ] [ PubMed ] [ Google Scholar ]
- 214. Connor AE, Baumgartner KB, Baumgartner RN, et al. (2016) Cigarette Smoking and Breast Cancer Risk in Hispanic and Non-Hispanic White Women: The Breast Cancer Health Disparities Study. J Womens Health 25:299–310. 10.1089/jwh.2015.5502 [ DOI ] [ PMC free article ] [ PubMed ] [ Google Scholar ]
- 215. Gera R, Mokbel R, Igor I, Mokbel K (2018) Does the Use of Hair Dyes Increase the Risk of Developing Breast Cancer? A Meta-analysis and Review of the Literature. Anticancer Res 38:707–716. 10.21873/anticanres.12276 [ DOI ] [ PubMed ] [ Google Scholar ]
- 216. Eberle CE, Sandler DP, Taylor KW, White AJ (2020) Hair dye and chemical straightener use and breast cancer risk in a large US population of black and white women. Int J Cancer 147:383–391. 10.1002/ijc.32738 [ DOI ] [ PMC free article ] [ PubMed ] [ Google Scholar ]
- 217. Cordina-Duverger E, Menegaux F, Popa A, et al. (2018) Night shift work and breast cancer: a pooled analysis of population-based case-control studies with complete work history. Eur J Epidemiol 33:369–379. 10.1007/s10654-018-0368-x [ DOI ] [ PubMed ] [ Google Scholar ]
- 218. Travis RC, Balkwill A, Fensom GK, et al. (2016) Night Shift Work and Breast Cancer Incidence: Three Prospective Studies and Meta-analysis of Published Studies. J Natl Cancer Inst 108:. 10.1093/jnci/djw169 [ DOI ] [ Google Scholar ]
- 219. Pahwa M, Labrèche F, Demers PA (2018) Night shift work and breast cancer risk: what do the meta-analyses tell us? Scand J Work Environ Health 44:432–435. 10.5271/sjweh.3738 [ DOI ] [ PubMed ] [ Google Scholar ]
- 220. Yang W-S, Deng Q, Fan W-Y, et al. (2014) Light exposure at night, sleep duration, melatonin, and breast cancer: a dose-response analysis of observational studies. Eur J Cancer Prev Off J Eur Cancer Prev Organ ECP 23:269–276. 10.1097/CEJ.0000000000000030 [ DOI ] [ PubMed ] [ Google Scholar ]
- 221. He C, Anand ST, Ebell MH, et al. (2015) Circadian disrupting exposures and breast cancer risk: a meta-analysis. Int Arch Occup Environ Health 88:533–547. 10.1007/s00420-014-0986-x [ DOI ] [ PubMed ] [ Google Scholar ]
- 222. Lu C, Sun H, Huang J, et al. (2017) Long-Term Sleep Duration as a Risk Factor for Breast Cancer: Evidence from a Systematic Review and Dose-Response Meta-Analysis. BioMed Res Int 2017:4845059. 10.1155/2017/4845059 [ DOI ] [ PMC free article ] [ PubMed ] [ Google Scholar ]
- 223. Qin Y, Zhou Y, Zhang X, et al. (2014) Sleep duration and breast cancer risk: a meta-analysis of observational studies. Int J Cancer 134:1166–1173. 10.1002/ijc.28452 [ DOI ] [ PubMed ] [ Google Scholar ]
- 224. Bahri N, Fathi Najafi T, Homaei Shandiz F, et al. (2019) The relation between stressful life events and breast cancer: a systematic review and meta-analysis of cohort studies. Breast Cancer Res Treat 176:53–61. 10.1007/s10549-019-05231-x [ DOI ] [ PubMed ] [ Google Scholar ]
- 225. Sergentanis TN, Zagouri F, Zografos GC (2010) Is antibiotic use a risk factor for breast cancer? A meta-analysis. Pharmacoepidemiol Drug Saf 19:1101–1107. 10.1002/pds.1986 [ DOI ] [ PubMed ] [ Google Scholar ]
- 226. Eom C-S, Park SM, Cho K-H (2012) Use of antidepressants and the risk of breast cancer: a meta-analysis. Breast Cancer Res Treat 136:635–645. 10.1007/s10549-012-2307-y [ DOI ] [ PubMed ] [ Google Scholar ]
- 227. Lu L, Shi L, Zeng J, Wen Z (2017) Aspirin as a potential modality for the chemoprevention of breast cancer: A dose-response meta-analysis of cohort studies from 857,831 participants. Oncotarget 8:40389–40401. 10.18632/oncotarget.16315 [ DOI ] [ PMC free article ] [ PubMed ] [ Google Scholar ]
- 228. Zhong S, Chen L, Zhang X, et al. (2015) Aspirin use and risk of breast cancer: systematic review and meta-analysis of observational studies. Cancer Epidemiol Biomark Prev Publ Am Assoc Cancer Res Cosponsored Am Soc Prev Oncol 24:1645–1655. 10.1158/1055-9965.EPI-15-0452 [ DOI ] [ PubMed ] [ Google Scholar ]
- 229. de Pedro M, Baeza S, Escudero M-T, et al. (2015) Effect of COX-2 inhibitors and other non-steroidal inflammatory drugs on breast cancer risk: a meta-analysis. Breast Cancer Res Treat 149:525–536. 10.1007/s10549-015-3267-9 [ DOI ] [ PubMed ] [ Google Scholar ]
- 230. Liu Y, Zhang X, Sun H, et al. (2019) Bisphosphonates and primary breast cancer risk: an updated systematic review and meta-analysis involving 963,995 women. Clin Epidemiol 11:593–603. 10.2147/CLEP.S194056 [ DOI ] [ PMC free article ] [ PubMed ] [ Google Scholar ]
- 231. Fournier A, Mesrine S, Gelot A, et al. (2017) Use of Bisphosphonates and Risk of Breast Cancer in a French Cohort of Postmenopausal Women. J Clin Oncol Off J Am Soc Clin Oncol 35:3230–3239. 10.1200/JCO.2016.71.4337 [ DOI ] [ PubMed ] [ Google Scholar ]
- 232. Rouach V, Goldshtein I, Buch A, et al. (2019) The association between adherence with oral bisphosphonates and the risk of breast cancer in post-menopausal women. J Bone Oncol 16:100202. 10.1016/j.jbo.2018.10.001 [ DOI ] [ PMC free article ] [ PubMed ] [ Google Scholar ]
- 233. Gennari A, Costa M, Puntoni M, et al. (2015) Breast cancer incidence after hormonal treatments for infertility: systematic review and meta-analysis of population-based studies. Breast Cancer Res Treat 150:405–413. 10.1007/s10549-015-3328-0 [ DOI ] [ PubMed ] [ Google Scholar ]
- 234. Sergentanis TN, Diamantaras A-A, Perlepe C, et al. (2014) IVF and breast cancer: a systematic review and meta-analysis. Hum Reprod Update 20:106–123. 10.1093/humupd/dmt034 [ DOI ] [ PubMed ] [ Google Scholar ]
- 235. Islam MM, Yang H-C, Nguyen P-A, et al. (2017) Exploring association between statin use and breast cancer risk: an updated meta-analysis. Arch Gynecol Obstet 296:1043–1053. 10.1007/s00404-017-4533-3 [ DOI ] [ PubMed ] [ Google Scholar ]
- 236. Colton T, Greenberg ER, Noller K, et al. (1993) Breast cancer in mothers prescribed diethylstilbestrol in pregnancy. Further follow-up. JAMA 269:2096–2100 [ PubMed ] [ Google Scholar ]
- 237. Greenberg ER, Barnes AB, Resseguie L, et al. (1984) Breast cancer in mothers given diethylstilbestrol in pregnancy. N Engl J Med 311:1393–1398. 10.1056/NEJM198411293112201 [ DOI ] [ PubMed ] [ Google Scholar ]
- 238. Hadjimichael OC, Meigs JW, Falcier FW, et al. (1984) Cancer risk among women exposed to exogenous estrogens during pregnancy. J Natl Cancer Inst 73:831–834 [ PubMed ] [ Google Scholar ]
- 239. Al Jishi T, Sergi C (2017) Current perspective of diethylstilbestrol (DES) exposure in mothers and offspring. Reprod Toxicol Elmsford N 71:71–77. 10.1016/j.reprotox.2017.04.009 [ DOI ] [ PubMed ] [ Google Scholar ]
- 240. Titus-Ernstoff L, Hatch EE, Hoover RN, et al. (2001) Long-term cancer risk in women given diethylstilbestrol (DES) during pregnancy. Br J Cancer 84:126–133. 10.1054/bjoc.2000.1521 [ DOI ] [ PMC free article ] [ PubMed ] [ Google Scholar ]
- 241. Palmer JR, Wise LA, Hatch EE, et al. (2006) Prenatal diethylstilbestrol exposure and risk of breast cancer. Cancer Epidemiol Biomark Prev Publ Am Assoc Cancer Res Cosponsored Am Soc Prev Oncol 15:1509–1514. 10.1158/1055-9965.EPI-06-0109 [ DOI ] [ PubMed ] [ Google Scholar ]
- 242. Troisi R, Hatch EE, Titus L, et al. (2019) Prenatal diethylstilbestrol exposure and cancer risk in women. Environ Mol Mutagen 60:395–403. 10.1002/em.22155 [ DOI ] [ PubMed ] [ Google Scholar ]
- 243. Cohn BA, La Merrill M, Krigbaum NY, et al. (2015) DDT Exposure in Utero and Breast Cancer. J Clin Endocrinol Metab 100:2865–2872. 10.1210/jc.2015-1841 [ DOI ] [ PMC free article ] [ PubMed ] [ Google Scholar ]
- 244. Tournaire M, Devouche E, Espié M, et al. (2015) Cancer Risk in Women Exposed to Diethylstilbestrol in Utero. Therapie 70:433–441. 10.2515/therapie/2015030 [ DOI ] [ PubMed ] [ Google Scholar ]
- 245. Guo J, Huang Y, Yang L, et al. (2015) Association between abortion and breast cancer: an updated systematic review and meta-analysis based on prospective studies. Cancer Causes Control CCC 26:811–819. 10.1007/s10552-015-0536-1 [ DOI ] [ PubMed ] [ Google Scholar ]
- 246. Deng Y, Xu H, Zeng X (2018) Induced abortion and breast cancer: An updated meta-analysis. Medicine (Baltimore) 97:e9613. 10.1097/MD.0000000000009613 [ DOI ] [ PMC free article ] [ PubMed ] [ Google Scholar ]
- 247. Beral V, Bull D, Doll R, et al. (2004) Breast cancer and abortion: collaborative reanalysis of data from 53 epidemiological studies, including 83?000 women with breast cancer from 16 countries. Lancet Lond Engl 363:1007–1016. 10.1016/S0140-6736(04)15835-2 [ DOI ] [ PubMed ] [ Google Scholar ]
- 248. Tong H, Wu Y, Yan Y, et al. (2020) No association between abortion and risk of breast cancer among nulliparous women. Medicine (Baltimore) 99:. 10.1097/MD.0000000000020251 [ DOI ] [ PMC free article ] [ PubMed ] [ Google Scholar ]
- 249. Salamat F, Niakan B, Keshtkar A, et al. (2018) Subtypes of Benign Breast Disease as a Risk Factor of Breast Cancer: A Systematic Review and Meta Analyses. Iran J Med Sci 43:355–364 [ PMC free article ] [ PubMed ] [ Google Scholar ]
- 250. Dyrstad SW, Yan Y, Fowler AM, Colditz GA (2015) Breast cancer risk associated with benign breast disease: systematic review and meta-analysis. Breast Cancer Res Treat 149:569–575. 10.1007/s10549-014-3254-6 [ DOI ] [ PubMed ] [ Google Scholar ]
- 251. Visser LL, Elshof LE, Schaapveld M, et al. (2018) Clinicopathological Risk Factors for an Invasive Breast Cancer Recurrence after Ductal Carcinoma In Situ-A Nested Case-Control Study. Clin Cancer Res Off J Am Assoc Cancer Res 24:3593–3601. 10.1158/1078-0432.CCR-18-0201 [ DOI ] [ PubMed ] [ Google Scholar ]
- 252. Zhang X, Dai H, Liu B, et al. (2016) Predictors for local invasive recurrence of ductal carcinoma in situ of the breast: a meta-analysis. Eur J Cancer Prev Off J Eur Cancer Prev Organ ECP 25:19–28. 10.1097/CEJ.0000000000000131 [ DOI ] [ PMC free article ] [ PubMed ] [ Google Scholar ]
- 253. Mannu GS, Wang Z, Broggio J, et al. (2020) Invasive breast cancer and breast cancer mortality after ductal carcinoma in situ in women attending for breast screening in England, 1988-2014: population based observational cohort study. BMJ 369:. 10.1136/bmj.m1570 [ DOI ] [ PMC free article ] [ PubMed ] [ Google Scholar ]
- 254. Dania V, Liu Y, Ademuyiwa F, et al. (2019) Associations of race and ethnicity with risk of developing invasive breast cancer after lobular carcinoma in situ. Breast Cancer Res BCR 21:120. 10.1186/s13058-019-1219-8 [ DOI ] [ PMC free article ] [ PubMed ] [ Google Scholar ]
- 255. Levi F, Randimbison L, Te V-C, La Vecchia C (2005) Invasive breast cancer following ductal and lobular carcinoma in situ of the breast. Int J Cancer 116:820–823. 10.1002/ijc.20870 [ DOI ] [ PubMed ] [ Google Scholar ]
- 256. Chuba PJ, Hamre MR, Yap J, et al. (2005) Bilateral risk for subsequent breast cancer after lobular carcinoma-in-situ: analysis of surveillance, epidemiology, and end results data. J Clin Oncol Off J Am Soc Clin Oncol 23:5534–5541. 10.1200/JCO.2005.04.038 [ DOI ] [ PubMed ] [ Google Scholar ]
- 257. McDivitt RW, Hutter RV, Foote FW, Stewart FW (1967) In situ lobular carcinoma. A prospective follow-up study indicating cumulative patient risks. JAMA 201:82–86. 10.1001/jama.201.2.82 [ DOI ] [ PubMed ] [ Google Scholar ]
- 258. King TA, Pilewskie M, Muhsen S, et al. (2015) Lobular Carcinoma in Situ: A 29-Year Longitudinal Experience Evaluating Clinicopathologic Features and Breast Cancer Risk. J Clin Oncol Off J Am Soc Clin Oncol 33:3945–3952. 10.1200/JCO.2015.61.4743 [ DOI ] [ PMC free article ] [ PubMed ] [ Google Scholar ]
- 259. Wong SM, King T, Boileau J-F, et al. (2017) Population-Based Analysis of Breast Cancer Incidence and Survival Outcomes in Women Diagnosed with Lobular Carcinoma In Situ. Ann Surg Oncol 24:2509–2517. 10.1245/s10434-017-5867-6 [ DOI ] [ PubMed ] [ Google Scholar ]
- 260. Page DL, Kidd TE, Dupont WD, et al. (1991) Lobular neoplasia of the breast: higher risk for subsequent invasive cancer predicted by more extensive disease. Hum Pathol 22:1232–1239. 10.1016/0046-8177(91)90105-x [ DOI ] [ PubMed ] [ Google Scholar ]
- 261. Sona MF, Myung S-K, Park K, Jargalsaikhan G (2018) Type 1 diabetes mellitus and risk of cancer: a meta-analysis of observational studies. Jpn J Clin Oncol 48:426–433. 10.1093/jjco/hyy047 [ DOI ] [ PubMed ] [ Google Scholar ]
- 262. Boyle P, Boniol M, Koechlin A, et al. (2012) Diabetes and breast cancer risk: a meta-analysis. Br J Cancer 107:1608–1617. 10.1038/bjc.2012.414 [ DOI ] [ PMC free article ] [ PubMed ] [ Google Scholar ]
- 263. Hope C, Robertshaw A, Cheung KL, et al. (2016) Relationship between HbA1c and cancer in people with or without diabetes: a systematic review. Diabet Med J Br Diabet Assoc 33:1013–1025. 10.1111/dme.13031 [ DOI ] [ PubMed ] [ Google Scholar ]
- 264. Xie C, Wang W, Li X, et al. (2019) Gestational diabetes mellitus and maternal breast cancer risk: a meta-analysis of the literature. J Matern-Fetal Neonatal Med Off J Eur Assoc Perinat Med Fed Asia Ocean Perinat Soc Int Soc Perinat Obstet 32:1022–1032. 10.1080/14767058.2017.1397117 [ DOI ] [ PubMed ] [ Google Scholar ]
- 265. Tang GH, Satkunam M, Pond GR, et al. (2018) Association of Metformin with Breast Cancer Incidence and Mortality in Patients with Type II Diabetes: A GRADE-Assessed Systematic Review and Meta-analysis. Cancer Epidemiol Biomark Prev Publ Am Assoc Cancer Res Cosponsored Am Soc Prev Oncol 27:627–635. 10.1158/1055-9965.EPI-17-0936 [ DOI ] [ PubMed ] [ Google Scholar ]
- 266. Guo M, Liu T, Li P, et al. (2019) Association Between Metabolic Syndrome and Breast Cancer Risk: An Updated Meta-Analysis of Follow-Up Studies. Front Oncol 9:1290. 10.3389/fonc.2019.01290 [ DOI ] [ PMC free article ] [ PubMed ] [ Google Scholar ]
- 267. Wu X, Wang M, Li S, Zhang Y (2016) Migraine and breast cancer risk: a meta-analysis of observational studies based on MOOSE compliant. Medicine (Baltimore) 95:e4031. 10.1097/MD.0000000000004031 [ DOI ] [ PMC free article ] [ PubMed ] [ Google Scholar ]
- 268. Winter AC, Rice MS, Fortner RT, et al. (2015) Migraine and breast cancer risk: a prospective cohort study and meta-analysis. J Natl Cancer Inst 107:381. 10.1093/jnci/dju381 [ DOI ] [ PMC free article ] [ PubMed ] [ Google Scholar ]
- 269. Preston DL, Kitahara CM, Freedman DM, et al. (2016) Breast cancer risk and protracted low-to-moderate dose occupational radiation exposure in the US Radiologic Technologists Cohort, 1983-2008. Br J Cancer 115:1105–1112. 10.1038/bjc.2016.292 [ DOI ] [ PMC free article ] [ PubMed ] [ Google Scholar ]
- 270. Rodgers KM, Udesky JO, Rudel RA, Brody JG (2018) Environmental chemicals and breast cancer: An updated review of epidemiological literature informed by biological mechanisms. Environ Res 160:152–182. 10.1016/j.envres.2017.08.045 [ DOI ] [ PubMed ] [ Google Scholar ]
- 271. Parada H, Gammon MD, Ettore HL, et al. (2019) Urinary concentrations of environmental phenols and their associations with breast cancer incidence and mortality following breast cancer. Environ Int 130:104890. 10.1016/j.envint.2019.05.084 [ DOI ] [ PMC free article ] [ PubMed ] [ Google Scholar ]
- 272. Trabert B, Falk RT, Figueroa JD, et al. (2014) Urinary bisphenol A-glucuronide and postmenopausal breast cancer in Poland. Cancer Causes Control CCC 25:1587–1593. 10.1007/s10552-014-0461-8 [ DOI ] [ PMC free article ] [ PubMed ] [ Google Scholar ]
- 273. Morgan M, Deoraj A, Felty Q, Roy D (2017) Environmental estrogen-like endocrine disrupting chemicals and breast cancer. Mol Cell Endocrinol 457:89–102. 10.1016/j.mce.2016.10.003 [ DOI ] [ PubMed ] [ Google Scholar ]
- 274. Ahern TP, Broe A, Lash TL, et al. (2019) Phthalate Exposure and Breast Cancer Incidence: A Danish Nationwide Cohort Study. J Clin Oncol Off J Am Soc Clin Oncol 37:1800–1809. 10.1200/JCO.18.02202 [ DOI ] [ PMC free article ] [ PubMed ] [ Google Scholar ]
- 275. Reeves KW, Díaz Santana M, Manson JE, et al. (2019) Urinary Phthalate Biomarker Concentrations and Postmenopausal Breast Cancer Risk. J Natl Cancer Inst 111:1059–1067. 10.1093/jnci/djz002 [ DOI ] [ PMC free article ] [ PubMed ] [ Google Scholar ]
- 276. Zuccarello P, Oliveri Conti G, Cavallaro F, et al. (2018) Implication of dietary phthalates in breast cancer. A systematic review. Food Chem Toxicol 118:667–674. 10.1016/j.fct.2018.06.011 [ DOI ] [ PubMed ] [ Google Scholar ]
- 277. Ghisari M, Long M, Røge DM, et al. (2017) Polymorphism in xenobiotic and estrogen metabolizing genes, exposure to perfluorinated compounds and subsequent breast cancer risk: A nested case-control study in the Danish National Birth Cohort. Environ Res 154:325–333. 10.1016/j.envres.2017.01.020 [ DOI ] [ PubMed ] [ Google Scholar ]
- 278. Mancini FR, Cano-Sancho G, Gambaretti J, et al. (2020) Perfluorinated alkylated substances serum concentration and breast cancer risk: Evidence from a nested case-control study in the French E3N cohort. Int J Cancer 146:917–928. 10.1002/ijc.32357 [ DOI ] [ PubMed ] [ Google Scholar ]
- 279. Hurley S, Goldberg D, Wang M, et al. (2018) Breast cancer risk and serum levels of per- and poly-fluoroalkyl substances: a case-control study nested in the California Teachers Study. Environ Health Glob Access Sci Source 17:83. 10.1186/s12940-018-0426-6 [ DOI ] [ PMC free article ] [ PubMed ] [ Google Scholar ]
- 280. Bae J-M, Kim EH (2016) Human papillomavirus infection and risk of breast cancer: a meta-analysis of case-control studies. Infect Agent Cancer 11:14. 10.1186/s13027-016-0058-9 [ DOI ] [ PMC free article ] [ PubMed ] [ Google Scholar ]
- 281. Farahmand M, Monavari SH, Shoja Z, et al. (2019) Epstein-Barr virus and risk of breast cancer: a systematic review and meta-analysis. Future Oncol Lond Engl 15:2873–2885. 10.2217/fon-2019-0232 [ DOI ] [ PubMed ] [ Google Scholar ]
- 282. Jansen LA, Backstein RM, Brown MH (2014) Breast size and breast cancer: a systematic review. J Plast Reconstr Aesthetic Surg JPRAS 67:1615–1623. 10.1016/j.bjps.2014.10.001 [ DOI ] [ PubMed ] [ Google Scholar ]
- 283. Manjer J, Kaaks R, Riboli E, Berglund G (2001) Risk of breast cancer in relation to anthropometry, blood pressure, blood lipids and glucose metabolism: a prospective study within the Malmö Preventive Project. Eur J Cancer Prev Off J Eur Cancer Prev Organ ECP 10:33–42. 10.1097/00008469-200102000-00004 [ DOI ] [ PubMed ] [ Google Scholar ]
- 284. Törnberg SA, Holm LE, Carstensen JM (1988) Breast cancer risk in relation to serum cholesterol, serum beta-lipoprotein, height, weight, and blood pressure. Acta Oncol Stockh Swed 27:31–37. 10.3109/02841868809090315 [ DOI ] [ PubMed ] [ Google Scholar ]
- 285. Yang Y, Lynch BM, Hodge AM, et al. (2017) Blood pressure and risk of breast cancer, overall and by subtypes: a prospective cohort study. J Hypertens 35:1371–1380. 10.1097/HJH.0000000000001372 [ DOI ] [ PubMed ] [ Google Scholar ]
- 286. Peeters PH, van Noord PA, Hoes AW, et al. (2000) Hypertension and breast cancer risk in a 19-year follow-up study (the DOM cohort). Diagnostic investigation into mammarian cancer. J Hypertens 18:249–254. 10.1097/00004872-200018030-00002 [ DOI ] [ PubMed ] [ Google Scholar ]
- 287. Agnoli C, Berrino F, Abagnato CA, et al. (2010) Metabolic syndrome and postmenopausal breast cancer in the ORDET cohort: a nested case-control study. Nutr Metab Cardiovasc Dis NMCD 20:41–48. 10.1016/j.numecd.2009.02.006 [ DOI ] [ PMC free article ] [ PubMed ] [ Google Scholar ]
- 288. Chen L, Malone KE, Li CI (2014) Bra wearing not associated with breast cancer risk: a population based case-control study. Cancer Epidemiol Biomark Prev Publ Am Assoc Cancer Res Cosponsored Am Soc Prev Oncol 23:2181–2185. 10.1158/1055-9965.EPI-14-0414 [ DOI ] [ PMC free article ] [ PubMed ] [ Google Scholar ]
- 289. Hsieh CC, Trichopoulos D (1991) Breast size, handedness and breast cancer risk. Eur J Cancer Oxf Engl 1990 27:131–135. 10.1016/0277-5379(91)90469-t [ DOI ] [ PubMed ] [ Google Scholar ]
- 290. Noels EC, Lapid O, Lindeman JHN, Bastiaannet E (2015) Breast implants and the risk of breast cancer: a meta-analysis of cohort studies. Aesthet Surg J 35:55–62. 10.1093/asj/sju006 [ DOI ] [ PubMed ] [ Google Scholar ]
- 291. Schüz J, Jacobsen R, Olsen JH, et al. (2006) Cellular Telephone Use and Cancer Risk: Update of a Nationwide Danish Cohort. JNCI J Natl Cancer Inst 98:1707–1713. 10.1093/jnci/djj464 [ DOI ] [ PubMed ] [ Google Scholar ]
- 292. McGrath KG (2003) An earlier age of breast cancer diagnosis related to more frequent use of antiperspirants/deodorants and underarm shaving. Eur J Cancer Prev Off J Eur Cancer Prev Organ ECP 12:479–485. 10.1097/00008469-200312000-00006 [ DOI ] [ PubMed ] [ Google Scholar ]
- 293. Fakri S, Al-Azzawi A, Al-Tawil N (2006) Antiperspirant use as a risk factor for breast cancer in Iraq. East Mediterr Health J Rev Sante Mediterr Orient Al-Majallah Al-Sihhiyah Li-Sharq Al-Mutawassit 12:478–482 [ PubMed ] [ Google Scholar ]
- 294. Mirick DK, Davis S, Thomas DB (2002) Antiperspirant use and the risk of breast cancer. J Natl Cancer Inst 94:1578–1580. 10.1093/jnci/94.20.1578 [ DOI ] [ PubMed ] [ Google Scholar ]
- 295. Krieger N (2015) Breast bruises and breast cancer. Breast Cancer Res 17:118. 10.1186/s13058-015-0631-y [ DOI ] [ PMC free article ] [ PubMed ] [ Google Scholar ]
- 296. Rigby JE, Morris JA, Lavelle J, et al. (2002) Can physical trauma cause breast cancer? Eur J Cancer Prev Off J Eur Cancer Prev Organ ECP 11:307–311. 10.1097/00008469-200206000-00014 [ DOI ] [ PubMed ] [ Google Scholar ]
- 297. Engel C, Fischer C (2015) Breast Cancer Risks and Risk Prediction Models. Breast Care 10:7–12. 10.1159/000376600 [ DOI ] [ PMC free article ] [ PubMed ] [ Google Scholar ]
- 298. Cintolo-Gonzalez JA, Braun D, Blackford AL, et al. (2017) Breast cancer risk models: a comprehensive overview of existing models, validation, and clinical applications. Breast Cancer Res Treat 164:263–284. 10.1007/s10549-017-4247-z [ DOI ] [ PubMed ] [ Google Scholar ]
- 299. Saslow D, Boetes C, Burke W, et al. (2007) American Cancer Society Guidelines for Breast Screening with MRI as an Adjunct to Mammography. CA Cancer J Clin 57:75–89. 10.3322/canjclin.57.2.75 [ DOI ] [ PubMed ] [ Google Scholar ]
- 300. Visvanathan K, Fabian CJ, Bantug E, et al. (2019) Use of Endocrine Therapy for Breast Cancer Risk Reduction: ASCO Clinical Practice Guideline Update. J Clin Oncol 37:3152–3165. 10.1200/JCO.19.01472 [ DOI ] [ PubMed ] [ Google Scholar ]
- 301. US Preventive Services Task Force, Owens DK, Davidson KW, et al. (2019) Risk Assessment, Genetic Counseling, and Genetic Testing for BRCA-Related Cancer: US Preventive Services Task Force Recommendation Statement. JAMA 322:652–665. 10.1001/jama.2019.10987 [ DOI ] [ PubMed ] [ Google Scholar ]
- 302. Tice JA, Bissell MCS, Miglioretti DL, et al. (2019) Validation of the breast cancer surveillance consortium model of breast cancer risk. Breast Cancer Res Treat 175:519–523. 10.1007/s10549-019-05167-2 [ DOI ] [ PMC free article ] [ PubMed ] [ Google Scholar ]
- 303. Vachon CM, Pankratz VS, Scott CG, et al. (2015) The contributions of breast density and common genetic variation to breast cancer risk. J Natl Cancer Inst 107:. 10.1093/jnci/dju397 [ DOI ] [ PMC free article ] [ PubMed ] [ Google Scholar ]
- 304. McCarthy AM, Guan Z, Welch M, et al. (2019) Performance of breast cancer risk assessment models in a large mammography cohort. J Natl Cancer Inst. 10.1093/jnci/djz177 [ DOI ] [ PMC free article ] [ PubMed ] [ Google Scholar ]
- 305. Ming C, Viassolo V, Probst-Hensch N, et al. (2019) Machine learning techniques for personalized breast cancer risk prediction: comparison with the BCRAT and BOADICEA models. Breast Cancer Res BCR 21:75. 10.1186/s13058-019-1158-4 [ DOI ] [ PMC free article ] [ PubMed ] [ Google Scholar ]
- 306. Gabrielson M, Ubhayasekera KA, Acharya SR, et al. (2020) Inclusion of Endogenous Plasma Dehydroepiandrosterone Sulfate and Mammographic Density in Risk Prediction Models for Breast Cancer. Cancer Epidemiol Biomark Prev Publ Am Assoc Cancer Res Cosponsored Am Soc Prev Oncol 29:574–581. 10.1158/1055-9965.EPI-19-1120 [ DOI ] [ PubMed ] [ Google Scholar ]
- 307. Wong EM, Southey MC, Terry MB (2020) Integrating DNA methylation measures to improve clinical risk assessment: are we there yet? The case of BRCA1 methylation marks to improve clinical risk assessment of breast cancer. Br J Cancer 122:1133–1140. 10.1038/s41416-019-0720-2 [ DOI ] [ PMC free article ] [ PubMed ] [ Google Scholar ]
- 308. Zhang X, Rice M, Tworoger SS, et al. (2018) Addition of a polygenic risk score, mammographic density, and endogenous hormones to existing breast cancer risk prediction models: A nested case-control study. PLoS Med 15:e1002644. 10.1371/journal.pmed.1002644 [ DOI ] [ PMC free article ] [ PubMed ] [ Google Scholar ]
- 309. Clendenen TV, Ge W, Koenig KL, et al. (2019) Breast cancer risk prediction in women aged 35-50 years: impact of including sex hormone concentrations in the Gail model. Breast Cancer Res BCR 21:42. 10.1186/s13058-019-1126-z [ DOI ] [ PMC free article ] [ PubMed ] [ Google Scholar ]
- 310. Shieh Y, Hu D, Ma L, et al. (2016) Breast cancer risk prediction using a clinical risk model and polygenic risk score. Breast Cancer Res Treat 159:513–525. 10.1007/s10549-016-3953-2 [ DOI ] [ PMC free article ] [ PubMed ] [ Google Scholar ]
- 311. Mavaddat N, Michailidou K, Dennis J, et al. (2019) Polygenic Risk Scores for Prediction of Breast Cancer and Breast Cancer Subtypes. Am J Hum Genet 104:21–34. 10.1016/j.ajhg.2018.11.002 [ DOI ] [ PMC free article ] [ PubMed ] [ Google Scholar ]
- 312. Rice MS, Tworoger SS, Hankinson SE, et al. (2017) Breast cancer risk prediction: an update to the Rosner-Colditz breast cancer incidence model. Breast Cancer Res Treat 166:227–240. 10.1007/s10549-017-4391-5 [ DOI ] [ PMC free article ] [ PubMed ] [ Google Scholar ]
- 313. Lécuyer L, Victor Bala A, Deschasaux M, et al. (2018) NMR metabolomic signatures reveal predictive plasma metabolites associated with long-term risk of developing breast cancer. Int J Epidemiol 47:484–494. 10.1093/ije/dyx271 [ DOI ] [ PubMed ] [ Google Scholar ]
- 314. Fung SM, Wong XY, Lee SX, et al. (2019) Performance of Single-Nucleotide Polymorphisms in Breast Cancer Risk Prediction Models: A Systematic Review and Meta-analysis. Cancer Epidemiol Biomark Prev Publ Am Assoc Cancer Res Cosponsored Am Soc Prev Oncol 28:506–521. 10.1158/1055-9965.EPI-18-0810 [ DOI ] [ PubMed ] [ Google Scholar ]
- 315. Hüsing A, Fortner RT, Kühn T, et al. (2017) Added Value of Serum Hormone Measurements in Risk Prediction Models for Breast Cancer for Women Not Using Exogenous Hormones: Results from the EPIC Cohort. Clin Cancer Res Off J Am Assoc Cancer Res 23:4181–4189. 10.1158/1078-0432.CCR-16-3011 [ DOI ] [ PubMed ] [ Google Scholar ]
- 316. Lee A, Mavaddat N, Wilcox AN, et al. (2019) BOADICEA: a comprehensive breast cancer risk prediction model incorporating genetic and nongenetic risk factors. Genet Med Off J Am Coll Med Genet 21:1708–1718. 10.1038/s41436-018-0406-9 [ DOI ] [ PMC free article ] [ PubMed ] [ Google Scholar ]
- 317. Colditz GA, Bohlke K, Berkey CS (2014) Breast cancer risk accumulation starts early: prevention must also. Breast Cancer Res Treat 145:567–579. 10.1007/s10549-014-2993-8 [ DOI ] [ PMC free article ] [ PubMed ] [ Google Scholar ]
- 318. Thomson CA, McCullough ML, Wertheim BC, et al. (2014) Nutrition and physical activity cancer prevention guidelines, cancer risk, and mortality in the women’s health initiative. Cancer Prev Res Phila Pa 7:42–53. 10.1158/1940-6207.CAPR-13-0258 [ DOI ] [ PMC free article ] [ PubMed ] [ Google Scholar ]
- 319. Rock CL, Thomson C, Gansler T, et al. American Cancer Society guideline for diet and physical activity for cancer prevention. CA Cancer J Clin n/a: 10.3322/caac.21591 [ DOI ] [ PubMed ] [ Google Scholar ]
- 320. Sauter ER (2018) Breast Cancer Prevention: Current Approaches and Future Directions. Eur J Breast Health 14:64–71. 10.5152/ejbh.2018.3978 [ DOI ] [ PMC free article ] [ PubMed ] [ Google Scholar ]
- 321. Anstey EH, Shoemaker ML, Barrera CM, et al. (2017) Breastfeeding and Breast Cancer Risk Reduction: Implications for Black Mothers. Am J Prev Med 53:S40–S46. 10.1016/j.amepre.2017.04.024 [ DOI ] [ PMC free article ] [ PubMed ] [ Google Scholar ]
- 322. Islami F, Liu Y, Jemal A, et al. (2015) Breastfeeding and breast cancer risk by receptor status--a systematic review and meta-analysis. Ann Oncol Off J Eur Soc Med Oncol 26:2398–2407. 10.1093/annonc/mdv379 [ DOI ] [ PMC free article ] [ PubMed ] [ Google Scholar ]
- 323. John EM, Hines LM, Phipps AI, et al. (2018) Reproductive history, breast-feeding and risk of triple negative breast cancer: The Breast Cancer Etiology in Minorities (BEM) study. Int J Cancer 142:2273–2285. 10.1002/ijc.31258 [ DOI ] [ PMC free article ] [ PubMed ] [ Google Scholar ]
- 324. Ma H, Ursin G, Xu X, et al. (2017) Reproductive factors and the risk of triple-negative breast cancer in white women and African-American women: a pooled analysis. Breast Cancer Res BCR 19:6. 10.1186/s13058-016-0799-9 [ DOI ] [ PMC free article ] [ PubMed ] [ Google Scholar ]
- 325. Fisher B, Costantino JP, Wickerham DL, et al. (2005) Tamoxifen for the prevention of breast cancer: current status of the National Surgical Adjuvant Breast and Bowel Project P-1 study. J Natl Cancer Inst 97:1652–1662. 10.1093/jnci/dji372 [ DOI ] [ PubMed ] [ Google Scholar ]
- 326. Martino S, Costantino J, McNabb M, et al. (2004) The role of selective estrogen receptor modulators in the prevention of breast cancer: comparison of the clinical trials. The Oncologist 9:116–125. 10.1634/theoncologist.9-2-116 [ DOI ] [ PubMed ] [ Google Scholar ]
- 327. Cuzick J, Sestak I, Thorat MA (2015) Impact of preventive therapy on the risk of breast cancer among women with benign breast disease. Breast Edinb Scotl 24 Suppl 2:S51–55. 10.1016/j.breast.2015.07.013 [ DOI ] [ PMC free article ] [ PubMed ] [ Google Scholar ]
- 328. Goss PE, Ingle JN, Alés-Martínez JE, et al. (2011) Exemestane for breast-cancer prevention in postmenopausal women. N Engl J Med 364:2381–2391. 10.1056/NEJMoa1103507 [ DOI ] [ PubMed ] [ Google Scholar ]
- 329. Cuzick J, Sestak I, Forbes JF, et al. (2014) Anastrozole for prevention of breast cancer in high-risk postmenopausal women (IBIS-II): an international, double-blind, randomised placebo-controlled trial. Lancet Lond Engl 383:1041–1048. 10.1016/S0140-6736(13)62292-8 [ DOI ] [ PubMed ] [ Google Scholar ]
- 330. Ropka ME, Keim J, Philbrick JT (2010) Patient decisions about breast cancer chemoprevention: a systematic review and meta-analysis. J Clin Oncol Off J Am Soc Clin Oncol 28:3090–3095. 10.1200/JCO.2009.27.8077 [ DOI ] [ PMC free article ] [ PubMed ] [ Google Scholar ]
- 331. Mocellin S, Pilati P, Briarava M, Nitti D (2016) Breast Cancer Chemoprevention: A Network Meta-Analysis of Randomized Controlled Trials. J Natl Cancer Inst 108:. 10.1093/jnci/djv318 [ DOI ] [ PubMed ] [ Google Scholar ]
- 332. Crew KD, Albain KS, Hershman DL, et al. (2017) How do we increase uptake of tamoxifen and other anti-estrogens for breast cancer prevention? NPJ Breast Cancer 3:20. 10.1038/s41523-017-0021-y [ DOI ] [ PMC free article ] [ PubMed ] [ Google Scholar ]
- 333. Cummings SR, Ensrud K, Delmas PD, et al. (2010) Lasofoxifene in postmenopausal women with osteoporosis. N Engl J Med 362:686–696. 10.1056/NEJMoa0808692 [ DOI ] [ PubMed ] [ Google Scholar ]
- 334. Metcalfe K, Eisen A, Senter L, et al. (2019) International trends in the uptake of cancer risk reduction strategies in women with a BRCA1 or BRCA2 mutation. Br J Cancer 121:15–21. 10.1038/s41416-019-0446-1 [ DOI ] [ PMC free article ] [ PubMed ] [ Google Scholar ]
- 335. Carbine NE, Lostumbo L, Wallace J, Ko H (2018) Risk-reducing mastectomy for the prevention of primary breast cancer. Cochrane Database Syst Rev 4:CD002748. 10.1002/14651858.CD002748.pub4 [ DOI ] [ PMC free article ] [ PubMed ] [ Google Scholar ]
- 336. Eleje GU, Eke AC, Ezebialu IU, et al. (2018) Risk-reducing bilateral salpingo-oophorectomy in women with BRCA1 or BRCA2 mutations. Cochrane Database Syst Rev 2018:. 10.1002/14651858.CD012464.pub2 [ DOI ] [ PMC free article ] [ PubMed ] [ Google Scholar ]
- 337. Jordan V, Khan M, Prill D (2019) Breast Cancer Screening: Why Can’t Everyone Agree? Prim Care 46:97–115. 10.1016/j.pop.2018.10.010 [ DOI ] [ PubMed ] [ Google Scholar ]
- 338. Monticciolo DL, Newell MS, Hendrick RE, et al. (2017) Breast Cancer Screening for Average-Risk Women: Recommendations From the ACR Commission on Breast Imaging. J Am Coll Radiol 14:1137–1143. 10.1016/j.jacr.2017.06.001 [ DOI ] [ PubMed ] [ Google Scholar ]
- 339. Bevers TB, Helvie M, Bonaccio E, et al. (2018) Breast Cancer Screening and Diagnosis, Version 3.2018, NCCN Clinical Practice Guidelines in Oncology. J Natl Compr Canc Netw 16:1362–1389. 10.6004/jnccn.2018.0083 [ DOI ] [ PubMed ] [ Google Scholar ]
- 340. Ebell MH, Thai TN, Royalty KJ (2018) Cancer screening recommendations: an international comparison of high income countries. Public Health Rev 39:7. 10.1186/s40985-018-0080-0 [ DOI ] [ PMC free article ] [ PubMed ] [ Google Scholar ]
- 341. Breast Cancer Screening. In: NCQA. https://www.ncqa.org/hedis/measures/breast-cancer-screening/ . Accessed 15 Jun 2020 [ Google Scholar ]
- 342. Nelson HD, Pappas M, Cantor A, et al. (2016) Harms of Breast Cancer Screening: Systematic Review to Update the 2009 U.S. Preventive Services Task Force Recommendation. Ann Intern Med 164:256–267. 10.7326/M15-0970 [ DOI ] [ PubMed ] [ Google Scholar ]
- 343. Nelson HD, Fu R, Cantor A, et al. (2016) Effectiveness of Breast Cancer Screening: Systematic Review and Meta-analysis to Update the 2009 U.S. Preventive Services Task Force Recommendation. Ann Intern Med 164:244–255. 10.7326/M15-0969 [ DOI ] [ PubMed ] [ Google Scholar ]
- 344. Harding C, Pompei F, Burmistrov D, Wilson R (2019) Long-term relationships between screening rates, breast cancer characteristics, and overdiagnosis in US counties, 1975-2009. Int J Cancer 144:476–488. 10.1002/ijc.31904 [ DOI ] [ PubMed ] [ Google Scholar ]
- 345. Vourtsis A, Berg WA (2019) Breast Density Implications and Supplemental Screening. Eur Radiol 29:1762–1777. 10.1007/s00330-018-5668-8 [ DOI ] [ PMC free article ] [ PubMed ] [ Google Scholar ]
- 346. Esserman LJ, WISDOM Study and Athena Investigators (2017) The WISDOM Study: breaking the deadlock in the breast cancer screening debate. NPJ Breast Cancer 3:34. 10.1038/s41523-017-0035-5 [ DOI ] [ PMC free article ] [ PubMed ] [ Google Scholar ]
- 347. (2017) TMIST Breast Screening Study - National Cancer Institute. https://www.cancer.gov/about-cancer/treatment/clinical-trials/nci-supported/tmist . Accessed 15 Jun 2020 [ Google Scholar ]
- 348. Berg WA (2016) Current Status of Supplemental Screening in Dense Breasts. J Clin Oncol Off J Am Soc Clin Oncol 34:1840–1843. 10.1200/JCO.2015.65.8674 [ DOI ] [ PMC free article ] [ PubMed ] [ Google Scholar ]
- 349. Assessment of Periodic Screening of Women With Denser Breast Using WBUS and DBT - Full Text View - ClinicalTrials.gov . https://clinicaltrials.gov/ct2/show/NCT02643966 . Accessed 15 Jun 2020
- 350. Turnbull C, Rahman N (2008) Genetic predisposition to breast cancer: past, present, and future. Annu Rev Genomics Hum Genet 9:321–345. 10.1146/annurev.genom.9.081307.164339 [ DOI ] [ PubMed ] [ Google Scholar ]
- 351. Kurian AW, Griffith KA, Hamilton AS, et al. (2017) Genetic Testing and Counseling Among Patients With Newly Diagnosed Breast Cancer. JAMA 317:531–534. 10.1001/jama.2016.16918 [ DOI ] [ PMC free article ] [ PubMed ] [ Google Scholar ]
- 352. Ripperger T, Gadzicki D, Meindl A, Schlegelberger B (2009) Breast cancer susceptibility: current knowledge and implications for genetic counselling. Eur J Hum Genet EJHG 17:722–731. 10.1038/ejhg.2008.212 [ DOI ] [ PMC free article ] [ PubMed ] [ Google Scholar ]
- 353. Slavin TP, Maxwell KN, Lilyquist J, et al. (2017) The contribution of pathogenic variants in breast cancer susceptibility genes to familial breast cancer risk. NPJ Breast Cancer 3:22. 10.1038/s41523-017-0024-8 [ DOI ] [ PMC free article ] [ PubMed ] [ Google Scholar ]
- 354. Tung N, Battelli C, Allen B, et al. (2015) Frequency of mutations in individuals with breast cancer referred for BRCA1 and BRCA2 testing using next-generation sequencing with a 25-gene panel. Cancer 121:25–33. 10.1002/cncr.29010 [ DOI ] [ PubMed ] [ Google Scholar ]
- 355. Hurley S, Goldberg D, Von Behren J, et al. (2011) Birth size and breast cancer risk among young California-born women. Cancer Causes Control CCC 22:1461–1470. 10.1007/s10552-011-9821-9 [ DOI ] [ PubMed ] [ Google Scholar ]
- 356. Michels KB, Xue F, Terry KL, Willett WC (2006) Longitudinal study of birthweight and the incidence of breast cancer in adulthood. Carcinogenesis 27:2464–2468. 10.1093/carcin/bgl105 [ DOI ] [ PubMed ] [ Google Scholar ]
- 357. Barber LE, Bertrand KA, Rosenberg L, et al. (2019) Pre- and perinatal factors and incidence of breast cancer in the Black Women’s Health Study. Cancer Causes Control CCC 30:87–95. 10.1007/s10552-018-1103-3 [ DOI ] [ PMC free article ] [ PubMed ] [ Google Scholar ]
- 358. Ma H, Ursin G, Xu X, et al. (2018) Body mass index at age 18 years and recent body mass index in relation to risk of breast cancer overall and ER/PR/HER2-defined subtypes in white women and African-American women: a pooled analysis. Breast Cancer Res BCR 20:5. 10.1186/s13058-017-0931-5 [ DOI ] [ PMC free article ] [ PubMed ] [ Google Scholar ]
- 359. Vrieling A, Buck K, Kaaks R, Chang-Claude J (2010) Adult weight gain in relation to breast cancer risk by estrogen and progesterone receptor status: a meta-analysis. Breast Cancer Res Treat 123:641–649. 10.1007/s10549-010-1116-4 [ DOI ] [ PubMed ] [ Google Scholar ]
- 360. Shieh Y, Scott CG, Jensen MR, et al. (2019) Body mass index, mammographic density, and breast cancer risk by estrogen receptor subtype. Breast Cancer Res BCR 21:48. 10.1186/s13058-019-1129-9 [ DOI ] [ PMC free article ] [ PubMed ] [ Google Scholar ]
- 361. Antoni S, Sasco AJ, dos Santos Silva I, McCormack V (2013) Is mammographic density differentially associated with breast cancer according to receptor status? A meta-analysis. Breast Cancer Res Treat 137:337–347. 10.1007/s10549-012-2362-4 [ DOI ] [ PubMed ] [ Google Scholar ]
- 362. Aktipis CA, Ellis BJ, Nishimura KK, Hiatt RA (2014) Modern reproductive patterns associated with estrogen receptor positive but not negative breast cancer susceptibility. Evol Med Public Health 2015:52–74. 10.1093/emph/eou028 [ DOI ] [ PMC free article ] [ PubMed ] [ Google Scholar ]
- 363. Anderson KN, Schwab RB, Martinez ME (2014) Reproductive risk factors and breast cancer subtypes: a review of the literature. Breast Cancer Res Treat 144:1–10. 10.1007/s10549-014-2852-7 [ DOI ] [ PMC free article ] [ PubMed ] [ Google Scholar ]
- 364. Unar-Munguía M, Torres-Mejía G, Colchero MA, González de Cosío T (2017) Breastfeeding Mode and Risk of Breast Cancer: A Dose-Response Meta-Analysis. J Hum Lact Off J Int Lact Consult Assoc 33:422–434. 10.1177/0890334416683676 [ DOI ] [ PubMed ] [ Google Scholar ]
- 365. Farvid MS, Eliassen AH, Cho E, et al. (2018) Dairy Consumption in Adolescence and Early Adulthood and Risk of Breast Cancer. Cancer Epidemiol Biomark Prev Publ Am Assoc Cancer Res Cosponsored Am Soc Prev Oncol 27:575–584. 10.1158/1055-9965.EPI-17-0345 [ DOI ] [ PMC free article ] [ PubMed ] [ Google Scholar ]
- 366. Genkinger JM, Makambi KH, Palmer JR, et al. (2013) Consumption of dairy and meat in relation to breast cancer risk in the Black Women’s Health Study. Cancer Causes Control CCC 24:675–684. 10.1007/s10552-013-0146-8 [ DOI ] [ PMC free article ] [ PubMed ] [ Google Scholar ]
- 367. McCullough ML, Rodriguez C, Diver WR, et al. (2005) Dairy, calcium, and vitamin D intake and postmenopausal breast cancer risk in the Cancer Prevention Study II Nutrition Cohort. Cancer Epidemiol Biomark Prev Publ Am Assoc Cancer Res Cosponsored Am Soc Prev Oncol 14:2898–2904. 10.1158/1055-9965.EPI-05-0611 [ DOI ] [ PubMed ] [ Google Scholar ]
- 368. Larsson SC, Bergkvist L, Wolk A (2009) Long-term meat intake and risk of breast cancer by oestrogen and progesterone receptor status in a cohort of Swedish women. Eur J Cancer Oxf Engl 1990 45:3042–3046. 10.1016/j.ejca.2009.04.035 [ DOI ] [ PubMed ] [ Google Scholar ]
- 369. Namazi N, Larijani B, Azadbakht L (2018) Association between the dietary inflammatory index and the incidence of cancer: a systematic review and meta-analysis of prospective studies. Public Health 164:148–156. 10.1016/j.puhe.2018.04.015 [ DOI ] [ PubMed ] [ Google Scholar ]
- 370. Jayedi A, Emadi A, Shab-Bidar S (2018) Dietary Inflammatory Index and Site-Specific Cancer Risk: A Systematic Review and Dose-Response Meta-Analysis. Adv Nutr Bethesda Md 9:388–403. 10.1093/advances/nmy015 [ DOI ] [ PMC free article ] [ PubMed ] [ Google Scholar ]
- 371. Moradi S, Issah A, Mohammadi H, Mirzaei K (2018) Associations between dietary inflammatory index and incidence of breast and prostate cancer: a systematic review and meta-analysis. Nutr Burbank Los Angel Cty Calif 55–56:168–178. 10.1016/j.nut.2018.04.018 [ DOI ] [ PubMed ] [ Google Scholar ]
- 372. Wang L, Liu C, Zhou C, et al. (2019) Meta-analysis of the association between the dietary inflammatory index (DII) and breast cancer risk. Eur J Clin Nutr 73:509–517. 10.1038/s41430-018-0196-9 [ DOI ] [ PubMed ] [ Google Scholar ]
- 373. Narita S, Inoue M, Saito E, et al. (2017) Dietary fiber intake and risk of breast cancer defined by estrogen and progesterone receptor status: the Japan Public Health Center-based Prospective Study. Cancer Causes Control CCC 28:569–578. 10.1007/s10552-017-0881-3 [ DOI ] [ PubMed ] [ Google Scholar ]
- 374. Ferrari P, Rinaldi S, Jenab M, et al. (2013) Dietary fiber intake and risk of hormonal receptor-defined breast cancer in the European Prospective Investigation into Cancer and Nutrition study. Am J Clin Nutr 97:344–353. 10.3945/ajcn.112.034025 [ DOI ] [ PubMed ] [ Google Scholar ]
- 375. Zhang C-X, Ho SC, Cheng S-Z, et al. (2011) Effect of dietary fiber intake on breast cancer risk according to estrogen and progesterone receptor status. Eur J Clin Nutr 65:929–936. 10.1038/ejcn.2011.57 [ DOI ] [ PubMed ] [ Google Scholar ]
- 376. Park Y, Brinton LA, Subar AF, et al. (2009) Dietary fiber intake and risk of breast cancer in postmenopausal women: the National Institutes of Health-AARP Diet and Health Study. Am J Clin Nutr 90:664–671. 10.3945/ajcn.2009.27758 [ DOI ] [ PMC free article ] [ PubMed ] [ Google Scholar ]
- 377. Suzuki R, Rylander-Rudqvist T, Ye W, et al. (2008) Dietary fiber intake and risk of postmenopausal breast cancer defined by estrogen and progesterone receptor status--a prospective cohort study among Swedish women. Int J Cancer 122:403–412. 10.1002/ijc.23060 [ DOI ] [ PubMed ] [ Google Scholar ]
- 378. Inoue-Choi M, Sinha R, Gierach GL, Ward MH (2016) Red and processed meat, nitrite, and heme iron intakes and postmenopausal breast cancer risk in the NIH-AARP Diet and Health Study. Int J Cancer 138:1609–1618. 10.1002/ijc.29901 [ DOI ] [ PMC free article ] [ PubMed ] [ Google Scholar ]
- 379. Graff RE, Cho E, Lindström S, et al. (2014) Premenopausal plasma ferritin levels, HFE polymorphisms, and risk of breast cancer in the nurses’ health study II. Cancer Epidemiol Biomark Prev Publ Am Assoc Cancer Res Cosponsored Am Soc Prev Oncol 23:516–524. 10.1158/1055-9965.EPI-13-0907 [ DOI ] [ PMC free article ] [ PubMed ] [ Google Scholar ]
- 380. Alexander DD, Morimoto LM, Mink PJ, Cushing CA (2010) A review and meta-analysis of red and processed meat consumption and breast cancer. Nutr Res Rev 23:349–365. 10.1017/S0954422410000235 [ DOI ] [ PubMed ] [ Google Scholar ]
- 381. Cho E, Chen WY, Hunter DJ, et al. (2006) Red meat intake and risk of breast cancer among premenopausal women. Arch Intern Med 166:2253–2259. 10.1001/archinte.166.20.2253 [ DOI ] [ PubMed ] [ Google Scholar ]
- 382. Linos E, Willett WC, Cho E, et al. (2008) Red meat consumption during adolescence among premenopausal women and risk of breast cancer. Cancer Epidemiol Biomark Prev Publ Am Assoc Cancer Res Cosponsored Am Soc Prev Oncol 17:2146–2151. 10.1158/1055-9965.EPI-08-0037 [ DOI ] [ PMC free article ] [ PubMed ] [ Google Scholar ]
- 383. Cui Y, Shikany JM, Liu S, et al. (2008) Selected antioxidants and risk of hormone receptor-defined invasive breast cancers among postmenopausal women in the Women’s Health Initiative Observational Study. Am J Clin Nutr 87:1009–1018. 10.1093/ajcn/87.4.1009 [ DOI ] [ PMC free article ] [ PubMed ] [ Google Scholar ]
- 384. Roswall N, Olsen A, Christensen J, et al. (2010) Micronutrient intake and breast cancer characteristics among postmenopausal women. Eur J Cancer Prev Off J Eur Cancer Prev Organ ECP 19:360–365. 10.1097/cej.0b013e32833ade68 [ DOI ] [ PubMed ] [ Google Scholar ]
- 385. Rosenberg L, Boggs DA, Bethea TN, et al. (2013) A prospective study of smoking and breast cancer risk among African-American women. Cancer Causes Control CCC 24:2207–2215. 10.1007/s10552-013-0298-6 [ DOI ] [ PMC free article ] [ PubMed ] [ Google Scholar ]
- 386. Kakugawa Y, Kawai M, Nishino Y, et al. (2015) Smoking and survival after breast cancer diagnosis in Japanese women: A prospective cohort study. Cancer Sci 106:1066–1074. 10.1111/cas.12716 [ DOI ] [ PMC free article ] [ PubMed ] [ Google Scholar ]
- 387. Tong J, Li Z, Shi J, et al. (2014) Passive smoking exposure from partners as a risk factor for ER+/PR+ double positive breast cancer in never-smoking Chinese urban women: a hospital-based matched case control study. PloS One 9:e97498. 10.1371/journal.pone.0097498 [ DOI ] [ PMC free article ] [ PubMed ] [ Google Scholar ]
- 388. Dossus L, Boutron-Ruault M-C, Kaaks R, et al. (2014) Active and passive cigarette smoking and breast cancer risk: results from the EPIC cohort. Int J Cancer 134:1871–1888. 10.1002/ijc.28508 [ DOI ] [ PubMed ] [ Google Scholar ]
- 389. Algra AM, Rothwell PM (2012) Effects of regular aspirin on long-term cancer incidence and metastasis: a systematic comparison of evidence from observational studies versus randomised trials. Lancet Oncol 13:518–527. 10.1016/S1470-2045(12)70112-2 [ DOI ] [ PubMed ] [ Google Scholar ]
- 390. Barnard ME, Boeke CE, Tamimi RM (2015) Established breast cancer risk factors and risk of intrinsic tumor subtypes. Biochim Biophys Acta 1856:73–85. 10.1016/j.bbcan.2015.06.002 [ DOI ] [ PubMed ] [ Google Scholar ]
- View on publisher site
- PDF (1.6 MB)
- Collections
Similar articles
Cited by other articles, links to ncbi databases.
- Download .nbib .nbib
- Format: AMA APA MLA NLM
Add to Collections

IMAGES
COMMENTS
This page highlights what's new and recent research in the detection and treatment of breast cancer. New methods to detect breast cancer, such as 3-D mammography, are described. Treatments tailored to breast cancer subtypes are discussed, including targeted therapies.
Breast Cancer Research is an online journal that publishes original research, reviews, editorials and reports on all aspects of breast cancer. Find out the latest articles on breast cancer risk factors, artificial intelligence, PDGFB, heparan sulfate, tamoxifen and more.
Breast Cancer Research Results and Study Updates See Advances in Breast Cancer Research for an overview of recent findings and progress, plus ongoing projects supported by NCI. Some Women Avoid Breast Cancer Screening After False-Positive Mammogram Results. Posted: October 4, 2024.
Dr. Leon-Ferre's research team recently identified a prognostic biomarker in patients diagnosed with triple-negative breast cancer (TNBC), a type of breast cancer that does not have estrogen or progesterone receptors and that does not have excessive expression of HER2. The researchers found that patients with high levels of naturally existing ...
Research studies. Current guidance on preventing and treating breast cancer as well as what might cause it (among other things) has come mainly from information discovered from research studies.Research studies can range from studies done in the lab to clinical trials done with hundreds of thousands of people.
Research can take decades to reach the bedside, but what discoveries are just around the corner for patients? Susan G. Komen shares all of this and more through Breast Cancer Breakthroughs, a virtual education series focusing on the new science and technology advancements that are poised to make a difference for patients in the near future.
Breast cancer is the most common cancer among women. It is estimated that 2.3 million new cases of BC are diagnosed globally each year. ... Bray F. Global Cancer Obser-Vatory: Cancer Today. International Agency for Research on Cancer; Lyon, France: 2020. [(accessed on 9 July 2021)]. Available online: https://gco.iarc.fr/today. [Google Scholar] 6.
Clinical trials are research studies that involve people. The clinical trials on this list are for breast cancer. All trials on the list are NCI-supported clinical trials, which are sponsored or otherwise financially supported by NCI. ... Women with ER positive breast cancer normally will receive endocrine therapy and some may receive ...
Breast cancerbreast cancer is a heterogeneous disease, with wide variation in tumour morphology, molecular characteristics, and clinical response. Invasive ductal carcinoma is the most common type of breast cancer, making up about 70% of tumours, and about 15-20% of tumours are invasive lobular carcinomas.
Breast cancer is the most commonly diagnosed invasive cancer among women both globally and within the United States and the number one cause of cancer ... Long J, Zheng W (2011) Genetic variants associated with breast-cancer risk: comprehensive research synopsis, meta-analysis, and epidemiological evidence. Lancet Oncol 12:477-488. 10. ...